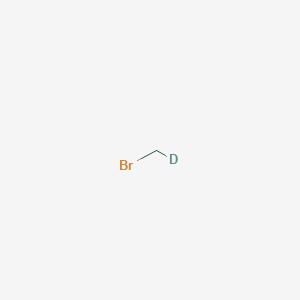
Bromo(deuterio)methane
描述
Bromo(deuterio)methane, also known as deuterated bromomethane, is a compound with the chemical formula CD2Br. It is a derivative of bromomethane (CH3Br) where one or more hydrogen atoms are replaced by deuterium, a stable isotope of hydrogen. This compound is of interest in various scientific fields due to its unique properties and applications.
准备方法
Synthetic Routes and Reaction Conditions: Bromo(deuterio)methane can be synthesized through several methods. One common approach involves the bromination of deuterated methane (CD4) using bromine (Br2) under ultraviolet light. The reaction proceeds as follows: [ \text{CD}_4 + \text{Br}_2 \rightarrow \text{CD}_3\text{Br} + \text{HBr} ]
Another method involves the reaction of deuterated methanol (CD3OH) with hydrogen bromide (HBr) in the presence of a catalyst. The reaction conditions typically include elevated temperatures and pressures to facilitate the substitution of the hydroxyl group with a bromine atom.
Industrial Production Methods: Industrial production of this compound often involves the large-scale bromination of deuterated methane. The process is carried out in specialized reactors equipped with ultraviolet light sources to ensure efficient and controlled bromination. The resulting product is then purified through distillation and other separation techniques to obtain high-purity this compound.
化学反应分析
Types of Reactions: Bromo(deuterio)methane undergoes various chemical reactions, including:
Substitution Reactions: It can participate in nucleophilic substitution reactions where the bromine atom is replaced by other nucleophiles such as hydroxide (OH-), cyanide (CN-), or amines (NH2-).
Elimination Reactions: Under basic conditions, this compound can undergo elimination reactions to form deuterated alkenes (CD2=CH2).
Addition Reactions: It can add to multiple bonds in the presence of catalysts, forming deuterated brominated compounds.
Common Reagents and Conditions:
Nucleophilic Substitution: Reagents such as sodium hydroxide (NaOH), potassium cyanide (KCN), and ammonia (NH3) are commonly used under mild to moderate temperatures.
Elimination Reactions: Strong bases like potassium tert-butoxide (KOtBu) are used under elevated temperatures.
Addition Reactions: Catalysts such as palladium or platinum are employed under controlled conditions.
Major Products:
Substitution Reactions: Deuterated alcohols (CD2OH), nitriles (CD2CN), and amines (CD2NH2).
Elimination Reactions: Deuterated alkenes (CD2=CH2).
Addition Reactions: Deuterated brominated compounds (CD2Br2).
科学研究应用
Medicinal Chemistry
Deuterated Drugs
The incorporation of deuterium in pharmaceuticals can significantly alter the metabolic and pharmacokinetic properties of drugs. For instance, deuterated compounds like deutetrabenazine have been approved by the FDA, demonstrating improved efficacy and reduced side effects compared to their non-deuterated counterparts . Bromo(deuterio)methane serves as a key reagent in synthesizing these deuterated drugs through various methods such as dehalogenation and hydrogen isotope exchange.
Kinetic Isotope Effects (KIE)
Deuterated compounds are essential for studying reaction mechanisms via KIE. The presence of deuterium alters the reaction rates, allowing researchers to infer details about transition states and pathways . this compound is frequently used as a standard in high-resolution mass spectrometry to investigate these effects.
Organic Synthesis
Electrochemical Deuteration
Recent advancements in electrochemical methods have highlighted the utility of this compound in organic synthesis. The electrochemical reduction of halides can lead to selective deuteration, enhancing atom economy and minimizing side reactions . For example, the use of this compound in electrocatalytic processes has shown promise for producing deuterated aromatic compounds efficiently .
Functionalization of Organic Compounds
this compound is also employed in the functionalization of various organic substrates. Its ability to serve as a source of deuterium makes it valuable for introducing deuterium labels into complex molecules, which is crucial for studying biological interactions and mechanisms .
Analytical Chemistry
Nuclear Magnetic Resonance (NMR) Spectroscopy
In NMR spectroscopy, deuterated solvents like this compound are essential for eliminating background signals from hydrogen. This enhances the clarity of spectral data, allowing for more accurate structural elucidation of compounds . The unique chemical shifts associated with deuterium provide additional information regarding molecular dynamics and interactions.
Mass Spectrometry
Deuterated compounds are widely used as internal standards in mass spectrometry. This compound's stable isotopic nature allows it to be utilized for calibrating instruments and quantifying analytes with high precision .
Case Studies
作用机制
The mechanism of action of bromo(deuterio)methane involves its participation in chemical reactions through the formation and breaking of bonds. The presence of deuterium affects the reaction kinetics and pathways due to the isotope effect, where the heavier deuterium atom leads to slower reaction rates compared to hydrogen. This property is exploited in studies of reaction mechanisms and in the design of deuterium-labeled compounds with improved stability.
相似化合物的比较
Bromo(deuterio)methane can be compared with other similar compounds such as:
Bromomethane (CH3Br): The non-deuterated analog of this compound, commonly used as a fumigant and in organic synthesis.
Bromoethane (C2H5Br): A related compound with an ethyl group instead of a methyl group, used in organic synthesis and as an alkylating agent.
Bromoform (CHBr3): A compound with three bromine atoms, used in organic synthesis and as a solvent.
Uniqueness: this compound is unique due to the presence of deuterium, which imparts distinct physical and chemical properties compared to its non-deuterated analogs. The isotope effect associated with deuterium makes it valuable in scientific research for studying reaction mechanisms and developing deuterium-labeled compounds with enhanced stability.
生物活性
Bromo(deuterio)methane, also known as bromoform when fully brominated, is a halogenated hydrocarbon with significant implications in biological systems, particularly in its role as a methanogenesis inhibitor and its potential environmental impacts. This article explores the biological activity of this compound, focusing on its mechanisms of action, effects on microbial communities, and potential applications in mitigating greenhouse gas emissions.
This compound is characterized by the substitution of hydrogen atoms with deuterium, which alters its physicochemical properties. The introduction of deuterium can influence the compound's lipophilicity and acidity, potentially affecting its biological interactions and metabolic pathways .
Inhibition of Methanogenesis
One of the primary biological activities of this compound is its inhibition of methanogenesis, a crucial process in the carbon cycle where methanogenic archaea convert organic matter into methane. This inhibition occurs through competitive interactions with key enzymes involved in the methanogenic pathway:
- Methyl-coenzyme M reductase (MCR) : This enzyme catalyzes the final step in methane production. This compound competes with substrates for binding, effectively reducing methane output .
- Coenzyme M methyltransferase : Similar to MCR, this enzyme is also inhibited by this compound, further disrupting methane synthesis pathways .
Case Studies and Experimental Findings
Several studies have investigated the efficacy of this compound as a methane inhibitor in livestock systems, particularly focusing on its application in ruminants.
Study 1: Cattle Trials
A notable trial involving cattle fed with Asparagopsis seaweed, which contains bromoform, reported significant reductions in methane production:
- Dosage : Cattle received doses ranging from 23.6 mg/day to 480 mg/day.
- Results : Methane production was reduced by 9% to 98%, with a notable long-term study showing a 28% reduction over 275 days at a dosage of 25 mg/kg dry matter .
Trial Duration | Dosage (mg/day) | Methane Reduction (%) |
---|---|---|
275 days | 25 | 28 |
Various | 23.6 - 480 | 9 - 98 |
Study 2: Environmental Impact Assessment
An environmental assessment highlighted that this compound's potential for mitigating methane emissions must be balanced against its ecological effects. The study indicated:
- Biodegradation : this compound exhibited only a 17% biodegradation rate over 28 days under aerobic conditions .
- Tissue Residues : Concerns were raised about the accumulation of bromoform residues in animal tissues and milk, necessitating further research into safe usage levels and regulatory compliance .
Research Findings on Microbial Communities
Research has also focused on how this compound affects microbial communities involved in methane production. A study examining flooded soils treated with various halogenated compounds found that while certain compounds did not significantly alter bacterial communities, they had profound effects on archaeal populations responsible for methanogenesis .
属性
IUPAC Name |
bromo(deuterio)methane | |
---|---|---|
Source | PubChem | |
URL | https://pubchem.ncbi.nlm.nih.gov | |
Description | Data deposited in or computed by PubChem | |
InChI |
InChI=1S/CH3Br/c1-2/h1H3/i1D | |
Source | PubChem | |
URL | https://pubchem.ncbi.nlm.nih.gov | |
Description | Data deposited in or computed by PubChem | |
InChI Key |
GZUXJHMPEANEGY-MICDWDOJSA-N | |
Source | PubChem | |
URL | https://pubchem.ncbi.nlm.nih.gov | |
Description | Data deposited in or computed by PubChem | |
Canonical SMILES |
CBr | |
Source | PubChem | |
URL | https://pubchem.ncbi.nlm.nih.gov | |
Description | Data deposited in or computed by PubChem | |
Isomeric SMILES |
[2H]CBr | |
Source | PubChem | |
URL | https://pubchem.ncbi.nlm.nih.gov | |
Description | Data deposited in or computed by PubChem | |
Molecular Formula |
CH3Br | |
Source | PubChem | |
URL | https://pubchem.ncbi.nlm.nih.gov | |
Description | Data deposited in or computed by PubChem | |
DSSTOX Substance ID |
DTXSID70584362 | |
Record name | Bromo(~2~H_1_)methane | |
Source | EPA DSSTox | |
URL | https://comptox.epa.gov/dashboard/DTXSID70584362 | |
Description | DSSTox provides a high quality public chemistry resource for supporting improved predictive toxicology. | |
Molecular Weight |
95.94 g/mol | |
Source | PubChem | |
URL | https://pubchem.ncbi.nlm.nih.gov | |
Description | Data deposited in or computed by PubChem | |
CAS No. |
1861-05-8 | |
Record name | Bromo(~2~H_1_)methane | |
Source | EPA DSSTox | |
URL | https://comptox.epa.gov/dashboard/DTXSID70584362 | |
Description | DSSTox provides a high quality public chemistry resource for supporting improved predictive toxicology. | |
Record name | 1861-05-8 | |
Source | European Chemicals Agency (ECHA) | |
URL | https://echa.europa.eu/information-on-chemicals | |
Description | The European Chemicals Agency (ECHA) is an agency of the European Union which is the driving force among regulatory authorities in implementing the EU's groundbreaking chemicals legislation for the benefit of human health and the environment as well as for innovation and competitiveness. | |
Explanation | Use of the information, documents and data from the ECHA website is subject to the terms and conditions of this Legal Notice, and subject to other binding limitations provided for under applicable law, the information, documents and data made available on the ECHA website may be reproduced, distributed and/or used, totally or in part, for non-commercial purposes provided that ECHA is acknowledged as the source: "Source: European Chemicals Agency, http://echa.europa.eu/". Such acknowledgement must be included in each copy of the material. ECHA permits and encourages organisations and individuals to create links to the ECHA website under the following cumulative conditions: Links can only be made to webpages that provide a link to the Legal Notice page. | |
体外研究产品的免责声明和信息
请注意,BenchChem 上展示的所有文章和产品信息仅供信息参考。 BenchChem 上可购买的产品专为体外研究设计,这些研究在生物体外进行。体外研究,源自拉丁语 "in glass",涉及在受控实验室环境中使用细胞或组织进行的实验。重要的是要注意,这些产品没有被归类为药物或药品,他们没有得到 FDA 的批准,用于预防、治疗或治愈任何医疗状况、疾病或疾病。我们必须强调,将这些产品以任何形式引入人类或动物的身体都是法律严格禁止的。遵守这些指南对确保研究和实验的法律和道德标准的符合性至关重要。