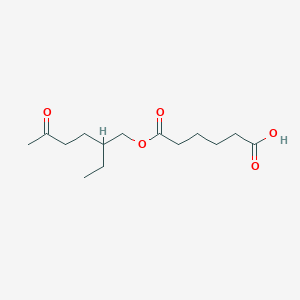
Mono(2-ethyl-5-oxohexyl) adipate
描述
Mono(2-ethyl-5-oxohexyl) adipate is an organic compound with the chemical formula C14H24O5. It is an ester derived from adipic acid and 2-ethyl-5-oxohexanol. This compound is known for its applications in various industrial and scientific fields due to its unique chemical properties.
准备方法
Synthetic Routes and Reaction Conditions
Mono(2-ethyl-5-oxohexyl) adipate can be synthesized through the esterification of adipic acid with 2-ethyl-5-oxohexanol. The reaction typically involves the use of a catalyst such as sulfuric acid or p-toluenesulfonic acid to facilitate the esterification process. The reaction is carried out under reflux conditions to ensure the complete conversion of the reactants to the desired ester.
Industrial Production Methods
In an industrial setting, the production of this compound involves large-scale esterification processes. The reactants, adipic acid and 2-ethyl-5-oxohexanol, are mixed in the presence of a catalyst and heated to the required temperature. The reaction mixture is then subjected to distillation to remove any unreacted starting materials and by-products, resulting in the pure ester.
化学反应分析
Types of Reactions
Mono(2-ethyl-5-oxohexyl) adipate undergoes various chemical reactions, including:
Oxidation: The compound can be oxidized to form corresponding carboxylic acids.
Reduction: Reduction reactions can convert the ester group to alcohols.
Substitution: The ester group can participate in nucleophilic substitution reactions.
Common Reagents and Conditions
Oxidation: Common oxidizing agents include potassium permanganate (KMnO4) and chromium trioxide (CrO3).
Reduction: Reducing agents such as lithium aluminum hydride (LiAlH4) and sodium borohydride (NaBH4) are used.
Substitution: Nucleophiles like hydroxide ions (OH-) and amines (NH2R) can be used in substitution reactions.
Major Products
Oxidation: Adipic acid and 2-ethyl-5-oxohexanoic acid.
Reduction: 2-ethyl-5-hydroxyhexanol and hexanediol.
Substitution: Various substituted esters and amides.
科学研究应用
Polymer Chemistry
Mono(2-ethyl-5-oxohexyl) adipate is primarily used as a plasticizer in polymer chemistry. Its incorporation into plastics enhances flexibility and durability, making it suitable for applications in products such as polyvinyl chloride (PVC). The compound's ability to lower the glass transition temperature of polymers allows for improved processing and performance characteristics in various environmental conditions.
Biological Research
In biological studies, this compound has been investigated for its metabolic pathways and potential effects on biological systems. Research indicates that it may influence metabolic processes, particularly in the liver, where it is formed during the metabolism of di(2-ethylhexyl) adipate (DEHA). Studies have shown that this compound can induce peroxisomal palmitoyl-CoA oxidation, a critical pathway for fatty acid metabolism.
Medical Applications
The compound is being explored for its potential in drug delivery systems due to its biocompatibility. Its structural characteristics allow it to be used in formulations that require controlled release of therapeutic agents, making it a candidate for developing new pharmaceutical applications.
Industrial Uses
In the industrial sector, this compound is utilized in the production of high-performance lubricants and coatings. Its properties contribute to enhanced performance metrics in lubricants, including improved viscosity and stability under varying temperatures.
Case Studies
Several case studies have highlighted the biological activity and safety profile of this compound:
- Human Biomonitoring Study : A controlled exposure study demonstrated that urinary excretion of metabolites peaked shortly after ingestion, indicating rapid metabolism and elimination from the body.
- Animal Toxicity Studies : Long-term studies on rodents revealed significant hepatic changes at high dietary levels of DEHA, from which this compound is derived.
- Physiologically Based Kinetic Modeling : Recent advancements in modeling have provided insights into how DEHA and its metabolites are processed in human physiology, aiding regulatory assessments for safe exposure levels.
作用机制
The mechanism of action of mono(2-ethyl-5-oxohexyl) adipate involves its interaction with various molecular targets and pathways. In biological systems, the compound is metabolized to its corresponding carboxylic acids and alcohols, which can then participate in further biochemical reactions. The ester group in the compound allows it to interact with enzymes and other proteins, influencing various cellular processes.
相似化合物的比较
Similar Compounds
Di(2-ethylhexyl) adipate (DEHA): A widely used plasticizer with similar chemical properties.
Mono(2-ethylhexyl) adipate: Another ester of adipic acid with comparable applications.
Di(2-ethylhexyl) phthalate (DEHP): A phthalate ester used as a plasticizer, but with different chemical structure and properties.
Uniqueness
Mono(2-ethyl-5-oxohexyl) adipate is unique due to its specific ester structure, which imparts distinct chemical and physical properties. Its ability to undergo various chemical reactions and its applications in multiple fields make it a valuable compound for scientific research and industrial use.
生物活性
Mono(2-ethyl-5-oxohexyl) adipate (5oxo-MEHA) is a metabolite derived from di(2-ethylhexyl) adipate (DEHA), a widely used plasticizer. This article explores the biological activity of 5oxo-MEHA, focusing on its metabolic pathways, toxicological effects, and potential health implications. The findings are supported by various studies and data tables summarizing key research outcomes.
Metabolism and Biokinetics
5oxo-MEHA is primarily formed through the metabolism of DEHA. Upon ingestion, DEHA undergoes hydrolysis to yield mono(2-ethylhexyl) adipate (MEHA) and adipic acid. The metabolic pathway is illustrated in the following table:
Substrate | Metabolite | Enzyme Activity | Source |
---|---|---|---|
Di(2-ethylhexyl) adipate | Mono(2-ethylhexyl) adipate (MEHA) | Hydrolysis | Takahashi et al. (1981) |
Mono(2-ethylhexyl) adipate | This compound (5oxo-MEHA) | Oxidation | Nehring et al. (2020) |
Mono(2-ethylhexyl) adipate | Adipic acid | Hydrolysis | Takahashi et al. (1981) |
In humans, studies indicate that 5oxo-MEHA accounts for approximately 0.45% of the ingested DEHA, suggesting limited conversion efficiency to this metabolite . The absorption and distribution of DEHA and its metabolites have been modeled using physiologically based pharmacokinetic (PBK) models, which help interpret the kinetics of these compounds in biological systems .
Toxicological Effects
Research has shown that high doses of DEHA can induce significant biological effects, including liver toxicity and peroxisome proliferation. A study conducted on Fischer 344 rats and B6C3F1 mice revealed that dietary concentrations of DEHA at or above 6000 mg/kg led to observable liver damage and increased liver weight due to peroxisomal proliferation . The following table summarizes key findings from toxicological studies:
Study | Species | Dose (mg/kg) | Effects Observed |
---|---|---|---|
National Toxicology Program (1981) | Rats | 6000+ | Liver hypertrophy, increased peroxisomal volume density |
Lake et al. (1997) | Mice | ≥1495 | Dose-dependent increases in liver weight and peroxisome proliferation |
Keith et al. (1992) | Rats | 0.5 - 4% in diet | Loss of glycogen in hepatocytes, increased catalase activity |
Health Implications
The potential health risks associated with exposure to 5oxo-MEHA are still under investigation. While some studies suggest that low levels of exposure may not pose significant risks, the long-term effects remain uncertain due to limited human data on chronic exposure . Moreover, the presence of metabolites like MEHA in urine has been used as a biomarker for exposure assessment in human populations .
Case Studies
Several case studies have highlighted the biological activity of 5oxo-MEHA and its parent compound DEHA:
- Human Biomonitoring Study : A study involving controlled exposure to DEHA showed that urinary excretion of its metabolites peaked within hours post-ingestion, indicating rapid metabolism and elimination from the body .
- Animal Toxicity Studies : Long-term studies on rodents have demonstrated that high dietary levels of DEHA can lead to significant hepatic changes, including hypertrophy and increased enzyme activity associated with fatty acid oxidation .
- Physiologically Based Kinetic Modeling : Recent advancements in PBK modeling have provided insights into how DEHA and its metabolites are processed in human physiology, aiding regulatory assessments for safe exposure levels .
属性
IUPAC Name |
6-(2-ethyl-5-oxohexoxy)-6-oxohexanoic acid | |
---|---|---|
Source | PubChem | |
URL | https://pubchem.ncbi.nlm.nih.gov | |
Description | Data deposited in or computed by PubChem | |
InChI |
InChI=1S/C14H24O5/c1-3-12(9-8-11(2)15)10-19-14(18)7-5-4-6-13(16)17/h12H,3-10H2,1-2H3,(H,16,17) | |
Source | PubChem | |
URL | https://pubchem.ncbi.nlm.nih.gov | |
Description | Data deposited in or computed by PubChem | |
InChI Key |
XLMWFRRVVDGMRV-UHFFFAOYSA-N | |
Source | PubChem | |
URL | https://pubchem.ncbi.nlm.nih.gov | |
Description | Data deposited in or computed by PubChem | |
Canonical SMILES |
CCC(CCC(=O)C)COC(=O)CCCCC(=O)O | |
Source | PubChem | |
URL | https://pubchem.ncbi.nlm.nih.gov | |
Description | Data deposited in or computed by PubChem | |
Molecular Formula |
C14H24O5 | |
Source | PubChem | |
URL | https://pubchem.ncbi.nlm.nih.gov | |
Description | Data deposited in or computed by PubChem | |
DSSTOX Substance ID |
DTXSID90928845 | |
Record name | 6-[(2-Ethyl-5-oxohexyl)oxy]-6-oxohexanoic acid | |
Source | EPA DSSTox | |
URL | https://comptox.epa.gov/dashboard/DTXSID90928845 | |
Description | DSSTox provides a high quality public chemistry resource for supporting improved predictive toxicology. | |
Molecular Weight |
272.34 g/mol | |
Source | PubChem | |
URL | https://pubchem.ncbi.nlm.nih.gov | |
Description | Data deposited in or computed by PubChem | |
CAS No. |
134998-72-4 | |
Record name | Mono(2-ethyl-5-oxohexyl) adipate | |
Source | ChemIDplus | |
URL | https://pubchem.ncbi.nlm.nih.gov/substance/?source=chemidplus&sourceid=0134998724 | |
Description | ChemIDplus is a free, web search system that provides access to the structure and nomenclature authority files used for the identification of chemical substances cited in National Library of Medicine (NLM) databases, including the TOXNET system. | |
Record name | 6-[(2-Ethyl-5-oxohexyl)oxy]-6-oxohexanoic acid | |
Source | EPA DSSTox | |
URL | https://comptox.epa.gov/dashboard/DTXSID90928845 | |
Description | DSSTox provides a high quality public chemistry resource for supporting improved predictive toxicology. | |
Retrosynthesis Analysis
AI-Powered Synthesis Planning: Our tool employs the Template_relevance Pistachio, Template_relevance Bkms_metabolic, Template_relevance Pistachio_ringbreaker, Template_relevance Reaxys, Template_relevance Reaxys_biocatalysis model, leveraging a vast database of chemical reactions to predict feasible synthetic routes.
One-Step Synthesis Focus: Specifically designed for one-step synthesis, it provides concise and direct routes for your target compounds, streamlining the synthesis process.
Accurate Predictions: Utilizing the extensive PISTACHIO, BKMS_METABOLIC, PISTACHIO_RINGBREAKER, REAXYS, REAXYS_BIOCATALYSIS database, our tool offers high-accuracy predictions, reflecting the latest in chemical research and data.
Strategy Settings
Precursor scoring | Relevance Heuristic |
---|---|
Min. plausibility | 0.01 |
Model | Template_relevance |
Template Set | Pistachio/Bkms_metabolic/Pistachio_ringbreaker/Reaxys/Reaxys_biocatalysis |
Top-N result to add to graph | 6 |
Feasible Synthetic Routes
体外研究产品的免责声明和信息
请注意,BenchChem 上展示的所有文章和产品信息仅供信息参考。 BenchChem 上可购买的产品专为体外研究设计,这些研究在生物体外进行。体外研究,源自拉丁语 "in glass",涉及在受控实验室环境中使用细胞或组织进行的实验。重要的是要注意,这些产品没有被归类为药物或药品,他们没有得到 FDA 的批准,用于预防、治疗或治愈任何医疗状况、疾病或疾病。我们必须强调,将这些产品以任何形式引入人类或动物的身体都是法律严格禁止的。遵守这些指南对确保研究和实验的法律和道德标准的符合性至关重要。