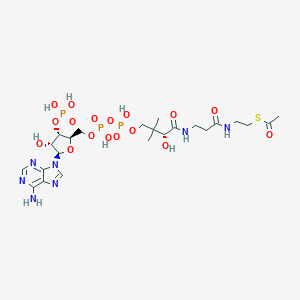
Acetyl coenzyme A
概述
描述
Metabolism: The Foundation of Life's Chemistry
Before delving into Acetyl CoA, it's crucial to understand the broader context of metabolism. Metabolism encompasses all the chemical reactions that occur within living organisms to maintain life. These intricate processes are broadly categorized into two main types:
- Catabolism: The breakdown of complex molecules into simpler ones, releasing energy in the process (e.g., cellular respiration).
- Anabolism: The synthesis of complex molecules from simpler precursors, requiring energy input (e.g., protein synthesis).
Defining Acetyl Coenzyme A: The Metabolic Crossroads
Acetyl CoA is a high-energy thioester molecule that plays a fundamental role in cellular metabolism. It is formed by the combination of an acetyl group (CH3CO-) and coenzyme A (CoA), a complex organic molecule derived from pantothenic acid (vitamin B5). The thioester bond between the acetyl group and CoA is a high-energy bond, meaning its hydrolysis releases a significant amount of free energy that can be used to drive other reactions.
The Structure of Acetyl CoA: A Molecular Perspective
Acetyl CoA's structure is crucial to its function. It comprises:
- Acetyl Group (CH3CO-): A two-carbon unit derived from various sources like carbohydrates, fats, and proteins.
- Coenzyme A (CoA): A complex molecule consisting of an adenosine diphosphate (ADP) moiety, pantothenic acid, and a β-mercaptoethylamine group. The terminal thiol (-SH) group of the β-mercaptoethylamine is the reactive site where the acetyl group binds, forming the thioester bond.
Component | Structure | Function |
Acetyl Group | CH3CO- | Provides the two-carbon unit for various metabolic reactions |
Coenzyme A (CoA) | Complex structure containing ADP, pantothenic acid, and β-mercaptoethylamine with a reactive thiol (-SH) group. | Acts as a carrier of acyl groups, facilitating their entry into metabolic pathways |
Thioester Bond | The bond between the acetyl group and the thiol group of CoA (CH3CO-S-CoA). This high-energy bond is crucial for driving reactions. | Stores and releases energy for metabolic reactions. |
The Importance of Acetyl CoA in Biochemical Pathways
Acetyl CoA's central role is evident in its involvement in numerous key metabolic pathways:
Citric Acid Cycle (Krebs Cycle/TCA Cycle):
- Acetyl CoA is the primary fuel for the citric acid cycle, a series of reactions occurring in the mitochondria that oxidize the acetyl group to carbon dioxide, generating high-energy electron carriers (NADH and FADH2) and a small amount of ATP.
- Research Finding: A study by Srere (1985) highlighted the importance of the citric acid cycle as the major pathway for ATP production in aerobic organisms, with Acetyl CoA playing a crucial entry point role.
Fatty Acid Metabolism:
- Fatty Acid Synthesis (Lipogenesis): Acetyl CoA is the precursor for fatty acid synthesis. In the cytoplasm, Acetyl CoA is carboxylated to malonyl CoA, the key regulatory step in fatty acid biosynthesis.
- Fatty Acid Oxidation (β-oxidation): Fatty acids are broken down in the mitochondria through β-oxidation, generating Acetyl CoA molecules that then enter the citric acid cycle.
- Case Study: Patients with carnitine deficiency have impaired fatty acid transport into the mitochondria, leading to reduced β-oxidation and decreased Acetyl CoA production, resulting in muscle weakness and other metabolic issues (Stanley, 2004).
Ketogenesis:
- During periods of prolonged fasting or in uncontrolled diabetes, when glucose availability is limited, the liver converts Acetyl CoA into ketone bodies (acetoacetate, β-hydroxybutyrate, and acetone), which can be used as an alternative fuel source by the brain and other tissues.
- Research Finding: Owen et al. (1967) demonstrated the critical role of ketone bodies as a fuel source for the brain during starvation, highlighting the importance of Acetyl CoA in this process.
Cholesterol Synthesis:
Acetyl CoA's Role in Energy Production and Biosynthesis
Acetyl CoA's involvement in both catabolic and anabolic pathways underscores its dual role:
- Energy Production: Through its entry into the citric acid cycle, Acetyl CoA is crucial for generating ATP, the cell's primary energy currency. The electrons carried by NADH and FADH2 produced in the citric acid cycle are used in oxidative phosphorylation to generate a large amount of ATP.
- Biosynthesis: Acetyl CoA serves as a building block for the synthesis of various essential molecules, including fatty acids, cholesterol, and certain amino acids.
Structure and Chemical Properties
Acetyl CoA is a high-energy thioester that serves as a crucial intermediate in numerous metabolic pathways. It is formed by the covalent bonding of an acetyl group to coenzyme A (CoA). This seemingly simple conjugation creates a molecule with remarkable chemical reactivity, enabling it to participate in diverse biochemical reactions.
Components of Acetyl CoA
Acetyl CoA comprises two main components:
Acetyl Group (Acetyl coenzyme A): This two-carbon unit is derived from various metabolic processes, including glycolysis (from pyruvate), fatty acid oxidation, and the breakdown of certain amino acids. It provides the carbon atoms that enter the citric acid cycle or are used in biosynthesis.
Coenzyme A (CoA or CoASH): This complex organic molecule is a derivative of pantothenic acid (vitamin B5). It consists of:
- Adenosine 3',5'-bisphosphate (ADP): A nucleotide component.
- Pantothenic Acid: A vitamin B5 derivative.
- β-Mercaptoethylamine: A thiol-containing group (-SH), which is the reactive site of CoA.
Chemical Bonds and Functional Groups
The key chemical features of Acetyl CoA are:
- Thiol Group (-SH): The thiol group on the β-mercaptoethylamine portion of CoA is crucial for its function. This group is highly nucleophilic, meaning it is attracted to positive charges and can readily form covalent bonds.
- Acetyl Group (this compound): This group contains a carbonyl group (C=O), which is also electrophilic (attracted to negative charges).
- Thioester Bond (C(O)-S): The crucial bond in Acetyl CoA is the thioester bond, formed between the carbonyl carbon of the acetyl group and the sulfur atom of the thiol group of CoA. This bond is a high-energy bond, meaning its hydrolysis releases a significant amount of free energy (approximately -31.4 kJ/mol or -7.5 kcal/mol). This energy release drives many metabolic reactions.
Role of the Thiol Group in Reactions
The thiol group of CoA is essential for several reasons:
- Nucleophilicity: The sulfur atom's high nucleophilicity allows it to readily attack electrophilic centers, such as the carbonyl carbon of the acetyl group or other acyl groups. This facilitates the formation and breaking of thioester bonds.
- Formation of Thioester Bonds: The thiol group is the site of thioester bond formation, a process that is crucial for activating acyl groups for subsequent reactions.
Formation of the Thioester Bond:
The formation of the thioester bond between the acetyl group and CoA is a condensation reaction, where a molecule of water is released. This reaction is typically coupled with an energy-releasing process, such as the hydrolysis of ATP, to make it thermodynamically favorable.
CH3COOH + HS-CoA + ATP → Acetyl coenzyme AS-CoA + AMP + PPi
(Acetic Acid) + (CoASH) + (ATP) → (Acetyl CoA) + (AMP) + (Pyrophosphate)
Chemical Properties and Reactivity:
The thioester bond in Acetyl CoA is less stable than a typical ester bond (C(O)-O) due to the larger size and lower electronegativity of sulfur compared to oxygen. This relative instability makes the thioester bond a high-energy bond. Hydrolysis of this bond releases a significant amount of free energy, which can be coupled to drive energetically unfavorable reactions.
Bond Type | Bond Energy (kJ/mol) | Relative Stability |
Ester | ~300-350 | More Stable |
Thioester | ~230-270 | Less Stable |
Research Finding: Jencks (1969) extensively studied the energetics of thioesters and highlighted their importance in driving biochemical reactions due to their high free energy of hydrolysis.
Case Study: The reaction catalyzed by citrate synthase in the citric acid cycle exemplifies the importance of the thioester bond. The hydrolysis of the thioester bond in Acetyl CoA provides the energy to drive the condensation of Acetyl CoA with oxaloacetate to form citrate. This reaction is highly exergonic (energy-releasing) and essential for the cycle's progression.
Acetyl Coenzyme a in Cellular Respiration
Linking Glycolysis to the Citric Acid Cycle: The Role of Acetyl CoA
Glycolysis, the initial stage of cellular respiration, occurs in the cytoplasm and involves the breakdown of glucose into two molecules of pyruvate. However, pyruvate cannot directly enter the citric acid cycle, which takes place in the mitochondrial matrix. This is where Acetyl CoA comes into play. Acetyl CoA acts as the crucial intermediate, bridging the gap between glycolysis and the citric acid cycle.
The Pyruvate Dehydrogenase Complex: The Gateway to Acetyl CoA Formation
The conversion of pyruvate to Acetyl CoA is catalyzed by the pyruvate dehydrogenase complex (PDC), a large multi-enzyme complex located in the mitochondrial matrix. This complex reaction is an oxidative decarboxylation, meaning it involves both oxidation (removal of electrons) and decarboxylation (release of carbon dioxide).
The PDC reaction can be summarized as follows:
Pyruvate + CoA + NAD+→ Acetyl CoA + CO2 + NADH + H+
The PDC is a complex of three enzymes:
- Pyruvate dehydrogenase (E1): Decarboxylates pyruvate, releasing CO2.
- Dihydrolipoyl transacetylase (E2): Transfers the acetyl group to CoA, forming Acetyl CoA.
- Dihydrolipoyl dehydrogenase (E3): Regenerates the oxidized form of lipoamide, a cofactor involved in the reaction.
Research Finding: Denton and Randle (1966) conducted extensive research on the regulation of pyruvate dehydrogenase, highlighting its importance in controlling the flow of carbon from glycolysis into the citric acid cycle.
Entry of Acetyl CoA into the Citric Acid Cycle (Krebs Cycle):
Once formed, Acetyl CoA enters the citric acid cycle (also known as the Krebs cycle or tricarboxylic acid cycle – TCA cycle) in the mitochondrial matrix. The first step of the cycle involves the condensation of Acetyl CoA with oxaloacetate, a four-carbon molecule, to form citrate, a six-carbon molecule. This reaction is catalyzed by citrate synthase.
Acetyl CoA + Oxaloacetate → Citrate + CoA
Oxidation of Acetyl CoA and Energy Production:
Within the citric acid cycle, the acetyl group of Acetyl CoA is completely oxidized to two molecules of CO2. This process generates high-energy electron carriers:
- NADH: Three molecules of NADH are produced per molecule of Acetyl CoA.
- FADH2: One molecule of FADH2 is produced per molecule of Acetyl CoA.
- GTP (or ATP): One molecule of GTP (which can be readily converted to ATP) is also produced.
Step in Citric Acid Cycle | Key Reaction | Products per Acetyl CoA |
1 | Acetyl CoA + Oxaloacetate → Citrate | Citrate |
3 | Isocitrate → α-Ketoglutarate | NADH, CO2 |
4 | α-Ketoglutarate → Succinyl CoA | NADH, CO2 |
5 | Succinyl CoA → Succinate | GTP/ATP |
6 | Succinate → Fumarate | FADH2 |
8 | Malate → Oxaloacetate | NADH |
Net per Acetyl CoA | 3 NADH, 1 FADH2, 1 GTP/ATP, 2 CO2 |
The NADH and FADH2produced in the citric acid cycle then donate their electrons to the electron transport chain (ETC) in the inner mitochondrial membrane. Through oxidative phosphorylation, the energy released during electron transfer is used to generate a large amount of ATP, the cell's primary energy currency.
Importance of the Citric Acid Cycle in Energy Production:
The citric acid cycle plays a pivotal role in cellular energy production:
- Generation of Reducing Equivalents: The cycle generates NADH and FADH2, which are essential for oxidative phosphorylation and ATP production.
- Central Metabolic Hub: The cycle also provides intermediates for the biosynthesis of other important molecules, such as amino acids and nucleotides.
Acetyl Coenzyme a in Fatty Acid Metabolism
Acetyl CoA in Fatty Acid Synthesis (Lipogenesis): The Building Block
Fatty acid synthesis, or lipogenesis, is the process by which the body synthesizes fatty acids from Acetyl CoA. This process primarily occurs in the liver, adipose tissue, and lactating mammary glands. Acetyl CoA serves as the fundamental building block, providing the two-carbon units that are sequentially added to the growing fatty acid chain.
Carboxylation of Acetyl CoA to Malonyl CoA: A Key Regulatory Step
The first committed step in fatty acid synthesis is the carboxylation of Acetyl CoA to malonyl CoA. This irreversible reaction is catalyzed by acetyl-CoA carboxylase (ACC), a biotin-dependent enzyme.
Acetyl CoA + CO2 + ATP → Malonyl CoA + ADP + Pi
This reaction is crucial for two main reasons:
- Commitment to Fatty Acid Synthesis: Malonyl CoA is specifically used for fatty acid synthesis and not other pathways, effectively committing the cell to this process.
- Regulation of Fatty Acid Oxidation: Malonyl CoA inhibits carnitine acyltransferase I (CAT-I), a key enzyme involved in fatty acid transport into the mitochondria for β-oxidation. This reciprocal regulation ensures that fatty acid synthesis and oxidation do not occur simultaneously.
Research Finding: Wakil (1989) extensively studied the structure and regulation of acetyl-CoA carboxylase, highlighting its crucial role in controlling fatty acid metabolism.
The Fatty Acid Synthase Complex:
The subsequent steps in fatty acid synthesis are catalyzed by the fatty acid synthase (FAS) complex, a large multi-enzyme complex. FAS sequentially adds two-carbon units (derived from malonyl CoA) to the growing fatty acyl chain, ultimately producing palmitate (a 16-carbon saturated fatty acid).
Acetyl CoA in Fatty Acid Oxidation (β-oxidation): The Energy Source
Fatty acid oxidation, or β-oxidation, is the process by which fatty acids are broken down in the mitochondria to generate Acetyl CoA, NADH, and FADH2. These products then enter the citric acid cycle and electron transport chain to produce ATP.
Breakdown of Fatty Acids to Generate Acetyl CoA:
β-oxidation occurs in a cyclical manner, with each cycle removing two carbon atoms from the fatty acyl chain in the form of Acetyl CoA. The process involves four main steps:
- Oxidation: Acyl-CoA dehydrogenase catalyzes the formation of a double bond between the α and β carbons of the fatty acyl CoA, producing FADH2.
- Hydration: Enoyl-CoA hydratase adds water across the double bond, forming a β-hydroxyacyl-CoA.
- Oxidation: β-Hydroxyacyl-CoA dehydrogenase oxidizes the β-hydroxy group to a ketone, producing NADH.
- Thiolysis: Thiolase cleaves the β-ketoacyl-CoA, releasing Acetyl CoA and a fatty acyl CoA shortened by two carbon atoms.
Case Study: Medium-chain acyl-CoA dehydrogenase (MCAD) deficiency is a common inherited metabolic disorder affecting β-oxidation. Individuals with this deficiency have impaired breakdown of medium-chain fatty acids, leading to accumulation of these fatty acids and potential episodes of hypoglycemia (Rinaldo et al., 1988).
Role of the Carnitine Shuttle in Fatty Acid Transport
Long-chain fatty acids cannot directly cross the inner mitochondrial membrane. They require a specialized transport system called the carnitine shuttle. This system involves three main steps:
- Activation: In the cytoplasm, fatty acids are activated by conversion to fatty acyl-CoA by acyl-CoA synthetase.
- Transport: Carnitine palmitoyltransferase I (CPT-I), located on the outer mitochondrial membrane, converts fatty acyl-CoA to fatty acylcarnitine. This molecule can then be transported across the inner mitochondrial membrane by carnitine acylcarnitine translocase.
- Regeneration: Inside the mitochondrial matrix, carnitine palmitoyltransferase II (CPT-II) converts fatty acylcarnitine back to fatty acyl-CoA, releasing carnitine. The fatty acyl-CoA can then undergo β-oxidation.
Research Finding: McGarry and Brown (1997) provided a comprehensive review of the carnitine palmitoyltransferase system and its role in fatty acid metabolism.
In Other Metabolic Pathways
SAcetyl CoA in Cholesterol Synthesis (Sterol Biosynthesis): A Key Precursor
Cholesterol, a vital component of cell membranes and a precursor for steroid hormones and bile acids, is synthesized through a complex pathway that begins with Acetyl CoA. This pathway occurs in the cytoplasm and endoplasmic reticulum of cells, primarily in the liver.
The initial steps of cholesterol synthesis involve:
- Formation of Acetoacetyl CoA: Two molecules of Acetyl CoA condense to form acetoacetyl CoA. This reaction is catalyzed by thiolase.
- Formation of HMG-CoA: Acetoacetyl CoA reacts with another molecule of Acetyl CoA to form β-hydroxy-β-methylglutaryl CoA (HMG-CoA). This reaction is catalyzed by HMG-CoA synthase.
- Formation of Mevalonate: HMG-CoA is then reduced to mevalonate by HMG-CoA reductase, a key regulatory enzyme in cholesterol biosynthesis. This step is the target of statin drugs, which lower cholesterol levels.
From mevalonate, a series of reactions leads to the formation of isopentenyl pyrophosphate, the building block for various isoprenoids, including cholesterol. Several isopentenyl pyrophosphate molecules condense to form squalene, which is then cyclized and further modified to produce cholesterol.
Acetyl CoA in Ketogenesis: An Alternative Fuel Source
Ketogenesis is the process by which the liver produces ketone bodies (acetoacetate, β-hydroxybutyrate, and acetone) from Acetyl CoA. This process occurs primarily during periods of prolonged fasting, starvation, or in uncontrolled diabetes, when glucose availability is limited. Ketone bodies serve as an alternative fuel source for the brain and other tissues that can utilize them.
The key steps in ketogenesis are:
- Formation of HMG-CoA: Similar to cholesterol synthesis, two molecules of Acetyl CoA condense to form acetoacetyl CoA, which then reacts with another Acetyl CoA to form HMG-CoA.
- Formation of β-Hydroxybutyrate and Acetone: Acetoacetate can be reduced to β-hydroxybutyrate by β-hydroxybutyrate dehydrogenase or spontaneously decarboxylated to acetone.
Case Study: In diabetic ketoacidosis (DKA), a severe complication of uncontrolled diabetes, the lack of insulin leads to increased fatty acid breakdown and overproduction of Acetyl CoA. This excess Acetyl CoA is shunted towards ketogenesis, leading to elevated levels of ketone bodies in the blood, causing acidosis (Kitabchi, Umpierrez, Miles, & Fisher, 2001).
Acetyl CoA in Amino Acid Metabolism
- Catabolism: The carbon skeletons of certain amino acids, such as leucine, isoleucine, and tryptophan, are degraded to Acetyl CoA or acetoacetyl CoA. These products can then enter the citric acid cycle for energy production or be used for other metabolic processes.
- Biosynthesis: While Acetyl CoA is not a direct precursor for most amino acids, it contributes indirectly by providing carbon atoms for the synthesis of certain non-essential amino acids through its role in the citric acid cycle, which generates precursors for amino acid biosynthesis.
Research Finding: Harper, Rodwell, and Mayes (1977) provided a comprehensive overview of amino acid metabolism, detailing the pathways by which amino acids are catabolized to various metabolic intermediates, including Acetyl CoA.
Significance of Acetyl CoA in These Pathways
Acetyl CoA's involvement in these pathways highlights its crucial role in:
- Lipid Metabolism: Connecting fatty acid metabolism with cholesterol synthesis and ketogenesis.
- Energy Homeostasis: Providing an alternative fuel source (ketone bodies) during periods of glucose scarcity.
- Interorgan Metabolic Communication: Facilitating the transport of energy (in the form of ketone bodies) from the liver to other tissues.
Regulation of Production and Utilization
Regulation of Acetyl CoA Production: The Pyruvate Dehydrogenase Complex
The primary source of Acetyl CoA for the citric acid cycle is the oxidative decarboxylation of pyruvate, catalyzed by the pyruvate dehydrogenase complex (PDC). The PDC is a major regulatory point in carbohydrate metabolism, and its activity is tightly controlled by various mechanisms.
Allosteric Regulation of Pyruvate Dehydrogenase
The PDC is subject to complex allosteric regulation:
- Activation:
- AMP: When cellular energy levels are low (high AMP/ATP ratio), AMP activates the PDC, promoting Acetyl CoA production to fuel ATP generation.
- CoA: High concentrations of CoA also activate the PDC, indicating a need for more Acetyl CoA.
- Ca2+: In muscle tissue, increased Ca2+ levels during muscle contraction activate the PDC, linking energy production to muscle activity.
- Inhibition:
- ATP: When cellular energy levels are high (low AMP/ATP ratio), ATP inhibits the PDC, reducing Acetyl CoA production.
- NADH: High NADH/NAD+ ratio, indicating an abundance of reducing equivalents, inhibits the PDC.
- Acetyl CoA: High levels of Acetyl CoA itself inhibit the PDC through product feedback inhibition.
Regulator | Effect on PDC Activity | Metabolic Significance |
AMP | Activation | Stimulates Acetyl CoA production when energy levels are low. |
ATP | Inhibition | Inhibits Acetyl CoA production when energy levels are high. |
NADH | Inhibition | Inhibits Acetyl CoA production when reducing equivalents are abundant. |
Acetyl CoA | Inhibition | Prevents overproduction of Acetyl CoA through product feedback. |
CoA | Activation | Stimulates Acetyl CoA production when CoA is available. |
Ca2+ | Activation (Muscle) | Links Acetyl CoA production to muscle contraction and energy demand in muscle tissue. |
Covalent Modification of Pyruvate Dehydrogenase:
The PDC is also regulated by covalent modification through phosphorylation and dephosphorylation.
- Phosphorylation (Inactivation): Pyruvate dehydrogenase kinase (PDK) phosphorylates the E1 subunit of the PDC, inactivating the complex. PDK is activated by ATP, NADH, and Acetyl CoA, reinforcing the allosteric inhibition.
- Dephosphorylation (Activation): Pyruvate dehydrogenase phosphatase (PDP) dephosphorylates the E1 subunit, activating the PDC. PDP is stimulated by Ca2+ and insulin.
Research Finding: Denton et al. (1972) extensively studied the regulation of pyruvate dehydrogenase by phosphorylation and dephosphorylation, demonstrating its importance in hormonal control of carbohydrate metabolism.
Influence of Energy Status (ATP/ADP Ratio, NADH/NAD+ Ratio):
The energy status of the cell, reflected by the ATP/ADP and NADH/NAD+ ratios, plays a crucial role in regulating Acetyl CoA levels.
- High ATP/ADP Ratio: Indicates high energy availability. This inhibits the PDC, reducing Acetyl CoA production and favoring energy storage.
- Low ATP/ADP Ratio: Indicates low energy availability. This activates the PDC, increasing Acetyl CoA production to fuel ATP generation through the citric acid cycle and oxidative phosphorylation.
- High NADH/NAD+ Ratio: Indicates an abundance of reducing equivalents. This inhibits the PDC, preventing further production of NADH and balancing redox status.
- Low NADH/NAD+Ratio: Indicates a need for reducing equivalents. This activates the PDC, increasing NADH production.
Hormonal Signals (Insulin, Glucagon):
- Insulin: In response to high blood glucose levels, insulin stimulates glucose uptake and glycolysis. It also activates PDP, leading to dephosphorylation and activation of the PDC, promoting Acetyl CoA production and favoring glucose oxidation.
- Glucagon: In response to low blood glucose levels, glucagon stimulates glycogen breakdown and gluconeogenesis. It also increases the activity of PDK, leading to phosphorylation and inactivation of the PDC, reducing Acetyl CoA production and favoring glucose sparing.
Case Study: In type 1 diabetes, the lack of insulin leads to decreased PDC activity, reduced glucose oxidation, and increased fatty acid breakdown. The excess Acetyl CoA generated from fatty acid oxidation is shunted towards ketogenesis, leading to ketoacidosis (Stacpoole, 1992).
Regulation of Acetyl CoA Utilization:
Clinical Significance and Research
Relevance of Acetyl CoA in Metabolic Disorders
Acetyl CoA's central role in carbohydrate, fat, and protein metabolism makes it a critical factor in metabolic health. Disruptions in Acetyl CoA metabolism can have profound consequences, contributing to the development of several metabolic disorders.
Conditions Where Acetyl CoA Metabolism is Disrupted
Diabetes Mellitus: In both type 1 and type 2 diabetes, Acetyl CoA metabolism is significantly affected.
- Type 1 Diabetes: The lack of insulin leads to decreased glucose uptake and utilization. Consequently, pyruvate dehydrogenase complex (PDC) activity is reduced, limiting the conversion of pyruvate to Acetyl CoA. This leads to increased fatty acid oxidation and the overproduction of Acetyl CoA from fatty acids, which is then shunted towards ketogenesis, resulting in diabetic ketoacidosis (DKA).
- Type 2 Diabetes: Insulin resistance impairs glucose utilization, while fatty acid oxidation is often elevated. This can lead to an accumulation of Acetyl CoA, contributing to insulin resistance and further metabolic dysfunction. Furthermore, mitochondrial dysfunction, often observed in type 2 diabetes, can impair the utilization of Acetyl CoA in the citric acid cycle.
Metabolic Syndrome: Metabolic syndrome, a cluster of conditions including abdominal obesity, high blood pressure, high blood sugar, and abnormal cholesterol levels, is closely linked to dysregulated Acetyl CoA metabolism. Insulin resistance, a hallmark of metabolic syndrome, leads to impaired glucose metabolism and increased fatty acid oxidation, resulting in elevated Acetyl CoA levels. This excess Acetyl CoA contributes to hepatic steatosis (fatty liver) and further exacerbates insulin resistance.
Non-Alcoholic Fatty Liver Disease (NAFLD) and Non-Alcoholic Steatohepatitis (NASH): In NAFLD and its more severe form, NASH, excessive accumulation of fat in the liver is a key characteristic. Dysregulation of Acetyl CoA metabolism plays a significant role in this process. Increased fatty acid oxidation and de novo lipogenesis (fatty acid synthesis from Acetyl CoA) contribute to the buildup of triglycerides in hepatocytes.
Inborn Errors of Metabolism: Several genetic disorders affect enzymes involved in Acetyl CoA metabolism, such as pyruvate dehydrogenase deficiency and medium-chain acyl-CoA dehydrogenase (MCAD) deficiency. These disorders can lead to severe metabolic complications, including lactic acidosis, hypoglycemia, and muscle weakness.
Metabolic Disorder | Key Metabolic Disruptions Related to Acetyl CoA | Clinical Manifestations |
Type 1 Diabetes | Decreased glucose utilization, increased fatty acid oxidation, overproduction of Acetyl CoA leading to ketogenesis. | Diabetic ketoacidosis (DKA), hyperglycemia. |
Type 2 Diabetes | Insulin resistance, impaired glucose utilization, increased fatty acid oxidation, mitochondrial dysfunction affecting Acetyl CoA utilization. | Hyperglycemia, dyslipidemia, increased risk of cardiovascular disease. |
Metabolic Syndrome | Insulin resistance, increased fatty acid oxidation, elevated Acetyl CoA levels, hepatic steatosis. | Abdominal obesity, hypertension, dyslipidemia, hyperglycemia. |
NAFLD/NASH | Increased fatty acid oxidation, de novo lipogenesis from Acetyl CoA, triglyceride accumulation in the liver. | Fatty liver, inflammation, potential progression to cirrhosis and liver cancer. |
Pyruvate Dehydrogenase Deficiency | Impaired conversion of pyruvate to Acetyl CoA, leading to decreased energy production from glucose. | Lactic acidosis, neurological problems. |
MCAD Deficiency | Impaired fatty acid oxidation, leading to accumulation of medium-chain fatty acids and decreased Acetyl CoA production during fasting. | Hypoglycemia, muscle weakness, potentially life-threatening metabolic crises. |
Ongoing Research Related to Acetyl CoA
Ongoing research is exploring the role of Acetyl CoA in various other disease states, including:
Cancer: Altered metabolism is a hallmark of cancer cells. Studies have shown that Acetyl CoA metabolism is often reprogrammed in cancer to support rapid cell growth and proliferation. Research is focused on understanding these metabolic changes and developing targeted therapies that disrupt Acetyl CoA metabolism in cancer cells.
Neurodegenerative Diseases: Emerging evidence suggests a link between impaired energy metabolism and neurodegenerative diseases like Alzheimer's and Parkinson's. Mitochondrial dysfunction and altered Acetyl CoA metabolism may contribute to neuronal damage and disease progression.
- Research Finding: Swerdlow (2012) discussed the role of mitochondrial dysfunction in neurodegenerative diseases, including potential impairments in Acetyl CoA metabolism.
Acetyl Coenzyme a and Biotechnology
Applications of Acetyl CoA in Biotechnology:
Acetyl CoA's central role in metabolism makes it a valuable target for biotechnological applications, primarily in the production of biofuels and other valuable bioproducts. Its involvement in various metabolic pathways allows for the synthesis of a wide range of compounds, including:
- Biofuels: Ethanol, butanol, and other alcohols.
- Organic Acids: Acetic acid, citric acid, lactic acid.
- Isoprenoids: Terpenes, carotenoids, and steroids.
- Polyketides: A diverse class of natural products with pharmaceutical applications.
Role of Acetyl CoA in Biofuel and Bioproduct Production:
- Ethanol Production: Acetyl CoA is a key intermediate in the production of ethanol through fermentation. Pyruvate, derived from glucose during glycolysis, is converted to Acetyl CoA, which is then reduced to acetaldehyde and subsequently to ethanol.
- Butanol Production: Butanol, a higher alcohol with desirable fuel properties, can also be produced from Acetyl CoA through a more complex pathway involving several enzymatic steps.
- Organic Acid Production: Various organic acids, such as acetic acid (vinegar) and citric acid (used as a food preservative and flavoring agent), can be produced from Acetyl CoA through specific metabolic pathways.
- Isoprenoid Production: Acetyl CoA is the precursor for the mevalonate pathway, which leads to the synthesis of isopentenyl pyrophosphate (IPP) and dimethylallyl pyrophosphate (DMAPP), the building blocks of isoprenoids. These compounds have diverse applications in pharmaceuticals, fragrances, and nutraceuticals.
Bioproduct | Pathway Involving Acetyl CoA | Biotechnological Significance |
---|---|---|
Ethanol | Pyruvate → Acetyl CoA → Acetaldehyde → Ethanol | Biofuel; widely used in alcoholic beverages and as a gasoline additive. |
Butanol | Complex pathway from Acetyl CoA involving several enzymes. | Advanced biofuel with superior properties compared to ethanol; potential for industrial applications. |
Acetic Acid | Acetyl CoA → Acetic Acid | Food preservative, flavoring agent, and industrial chemical. |
Citric Acid | Citric acid cycle (Acetyl CoA is the entry point). | Food preservative, flavoring agent, and industrial chemical. |
Isoprenoids | Acetyl CoA → Mevalonate pathway → IPP/DMAPP → Isoprenoids | Pharmaceuticals (e.g., statins), fragrances, nutraceuticals (e.g., carotenoids). |
Metabolic Engineering to Manipulate Acetyl CoA Pathways:
Metabolic engineering involves the targeted modification of metabolic pathways to enhance the production of desired compounds. Several strategies are employed to manipulate Acetyl CoA pathways:
Overexpression of Key Enzymes: Increasing the expression of enzymes involved in the production of Acetyl CoA or its conversion to desired products can enhance the flux through these pathways. For example, overexpressing pyruvate dehydrogenase complex (PDC) can increase Acetyl CoA production from pyruvate.
Knockout or Downregulation of Competing Pathways: Blocking or reducing the activity of competing pathways that consume Acetyl CoA can redirect metabolic flux towards the desired product. For example, downregulating enzymes involved in fatty acid synthesis can increase the availability of Acetyl CoA for other pathways.
Heterologous Expression of Genes: Introducing genes from other organisms that encode enzymes with desired activities can create new metabolic pathways or improve existing ones. For example, introducing genes for butanol biosynthesis into a microorganism can enable it to produce butanol from Acetyl CoA.
Flux Balancing Analysis: Mathematical modeling techniques, such as flux balance analysis (FBA), can be used to predict the optimal metabolic fluxes for maximizing product yield and identify bottlenecks in the pathway.
Case Study: Researchers have successfully engineered Escherichia coli to produce high titers of biofuels like ethanol and butanol by manipulating Acetyl CoA pathways. For example, by overexpressing PDC and introducing genes for butanol biosynthesis, they achieved significant improvements in butanol production (Atsumi et al., 2008).
Frequently Asked Questions (FAQs) about Acetyl Coenzyme A
Q1: What is the main function of Acetyl CoA?
Acetyl CoA plays a central role in metabolism, acting as a crucial link between several key metabolic pathways. Its primary functions include:
- Linking Glycolysis to the Citric Acid Cycle: Acetyl CoA is the primary fuel that enters the citric acid cycle (Krebs cycle), the central metabolic pathway for energy production in aerobic organisms. It connects the breakdown of glucose (glycolysis) with the complete oxidation of carbon fuels to CO2.
- Involvement in Fatty Acid Metabolism: Acetyl CoA plays a dual role in fatty acid metabolism. It is the building block for fatty acid synthesis (lipogenesis) and the product of fatty acid breakdown (β-oxidation).
- Participation in other metabolic pathways: Acetyl CoA is also involved in ketogenesis (the production of ketone bodies), cholesterol synthesis, and the metabolism of certain amino acids.
Q2: Where is Acetyl CoA produced in the cell?
Acetyl CoA is produced in different cellular compartments depending on the metabolic pathway:
- Mitochondria: The majority of Acetyl CoA is produced in the mitochondrial matrix through the oxidative decarboxylation of pyruvate, catalyzed by the pyruvate dehydrogenase complex (PDC). This is the primary source of Acetyl CoA for the citric acid cycle. Beta oxidation also occurs in the mitochondria.
- Cytoplasm: Acetyl CoA is also produced in the cytoplasm, primarily for fatty acid synthesis. Cytoplasmic Acetyl CoA is generated from mitochondrial Acetyl CoA through the citrate shuttle. Citrate, formed in the mitochondria, is transported to the cytoplasm, where it is cleaved by ATP citrate lyase to generate Acetyl CoA and oxaloacetate.
This compartmentalization allows for the regulation of different metabolic processes in distinct cellular environments.
Q3: What is the relationship between Acetyl CoA and ATP?
- Citric Acid Cycle: The oxidation of the acetyl group of Acetyl CoA in the citric acid cycle generates high-energy electron carriers NADH and FADH2, as well as one molecule of GTP (which is readily converted to ATP).
- Oxidative Phosphorylation: The NADH and FADH2 produced in the citric acid cycle donate their electrons to the electron transport chain (ETC) in the inner mitochondrial membrane. The energy released during electron transfer is used to generate a proton gradient across the membrane, which drives ATP synthesis by ATP synthase.
Therefore, Acetyl CoA is essential for the efficient production of ATP, the cell's primary energy currency.
Q4: How is Acetyl CoA involved in diabetes?
Dysregulation of Acetyl CoA metabolism is frequently observed in diabetes, impacting both glucose and lipid metabolism.
- Type 1 Diabetes: The lack of insulin leads to decreased glucose uptake and utilization. This results in reduced activity of the pyruvate dehydrogenase complex (PDC), the enzyme complex that converts pyruvate to Acetyl CoA. Consequently, the body relies more heavily on fatty acid oxidation for energy production. This leads to an overproduction of Acetyl CoA from fatty acids, which is then shunted towards ketogenesis, resulting in the accumulation of ketone bodies and potentially leading to diabetic ketoacidosis (DKA).
- Type 2 Diabetes: Insulin resistance, a hallmark of type 2 diabetes, impairs glucose metabolism and often leads to increased fatty acid oxidation. This can result in elevated levels of Acetyl CoA, which can contribute to further insulin resistance and other metabolic complications. Mitochondrial dysfunction, often associated with type 2 diabetes, can also impair the utilization of Acetyl CoA in the citric acid cycle.
In both types of diabetes, the dysregulation of Acetyl CoA metabolism contributes to the complex metabolic abnormalities observed in the disease.
Q5: What is the difference between CoA and Acetyl CoA?
CoA (Coenzyme A) and Acetyl CoA are chemically distinct molecules with different roles.
- CoA (CoASH): CoA is a complex organic molecule derived from pantothenic acid (vitamin B5). It consists of an adenosine diphosphate (ADP) moiety, pantothenic acid, and a β-mercaptoethylamine group. The terminal thiol (-SH) group of the β-mercaptoethylamine is the reactive site. CoA acts as a carrier of acyl groups.
- Acetyl CoA (Acetyl coenzyme AS-CoA): Acetyl CoA is formed when an acetyl group (this compound) is attached to the thiol group of CoA through a thioester bond. This bond is a high-energy bond, and its hydrolysis releases energy that can drive other reactions. Acetyl CoA is an activated form of acetate and plays a crucial role in various metabolic pathways.
属性
IUPAC Name |
S-[2-[3-[[(2R)-4-[[[(2R,3S,4R,5R)-5-(6-aminopurin-9-yl)-4-hydroxy-3-phosphonooxyoxolan-2-yl]methoxy-hydroxyphosphoryl]oxy-hydroxyphosphoryl]oxy-2-hydroxy-3,3-dimethylbutanoyl]amino]propanoylamino]ethyl] ethanethioate | |
---|---|---|
Source | PubChem | |
URL | https://pubchem.ncbi.nlm.nih.gov | |
Description | Data deposited in or computed by PubChem | |
InChI |
InChI=1S/C23H38N7O17P3S/c1-12(31)51-7-6-25-14(32)4-5-26-21(35)18(34)23(2,3)9-44-50(41,42)47-49(39,40)43-8-13-17(46-48(36,37)38)16(33)22(45-13)30-11-29-15-19(24)27-10-28-20(15)30/h10-11,13,16-18,22,33-34H,4-9H2,1-3H3,(H,25,32)(H,26,35)(H,39,40)(H,41,42)(H2,24,27,28)(H2,36,37,38)/t13-,16-,17-,18+,22-/m1/s1 | |
Source | PubChem | |
URL | https://pubchem.ncbi.nlm.nih.gov | |
Description | Data deposited in or computed by PubChem | |
InChI Key |
ZSLZBFCDCINBPY-ZSJPKINUSA-N | |
Source | PubChem | |
URL | https://pubchem.ncbi.nlm.nih.gov | |
Description | Data deposited in or computed by PubChem | |
Canonical SMILES |
CC(=O)SCCNC(=O)CCNC(=O)C(C(C)(C)COP(=O)(O)OP(=O)(O)OCC1C(C(C(O1)N2C=NC3=C(N=CN=C32)N)O)OP(=O)(O)O)O | |
Source | PubChem | |
URL | https://pubchem.ncbi.nlm.nih.gov | |
Description | Data deposited in or computed by PubChem | |
Isomeric SMILES |
CC(=O)SCCNC(=O)CCNC(=O)[C@@H](C(C)(C)COP(=O)(O)OP(=O)(O)OC[C@@H]1[C@H]([C@H]([C@@H](O1)N2C=NC3=C(N=CN=C32)N)O)OP(=O)(O)O)O | |
Source | PubChem | |
URL | https://pubchem.ncbi.nlm.nih.gov | |
Description | Data deposited in or computed by PubChem | |
Molecular Formula |
C23H38N7O17P3S | |
Source | PubChem | |
URL | https://pubchem.ncbi.nlm.nih.gov | |
Description | Data deposited in or computed by PubChem | |
DSSTOX Substance ID |
DTXSID30992686 | |
Record name | Acetyl CoA | |
Source | EPA DSSTox | |
URL | https://comptox.epa.gov/dashboard/DTXSID30992686 | |
Description | DSSTox provides a high quality public chemistry resource for supporting improved predictive toxicology. | |
Molecular Weight |
809.6 g/mol | |
Source | PubChem | |
URL | https://pubchem.ncbi.nlm.nih.gov | |
Description | Data deposited in or computed by PubChem | |
Physical Description |
Solid | |
Record name | Acetyl-CoA | |
Source | Human Metabolome Database (HMDB) | |
URL | http://www.hmdb.ca/metabolites/HMDB0001206 | |
Description | The Human Metabolome Database (HMDB) is a freely available electronic database containing detailed information about small molecule metabolites found in the human body. | |
Explanation | HMDB is offered to the public as a freely available resource. Use and re-distribution of the data, in whole or in part, for commercial purposes requires explicit permission of the authors and explicit acknowledgment of the source material (HMDB) and the original publication (see the HMDB citing page). We ask that users who download significant portions of the database cite the HMDB paper in any resulting publications. | |
CAS No. |
72-89-9 | |
Record name | Acetyl CoA | |
Source | CAS Common Chemistry | |
URL | https://commonchemistry.cas.org/detail?cas_rn=72-89-9 | |
Description | CAS Common Chemistry is an open community resource for accessing chemical information. Nearly 500,000 chemical substances from CAS REGISTRY cover areas of community interest, including common and frequently regulated chemicals, and those relevant to high school and undergraduate chemistry classes. This chemical information, curated by our expert scientists, is provided in alignment with our mission as a division of the American Chemical Society. | |
Explanation | The data from CAS Common Chemistry is provided under a CC-BY-NC 4.0 license, unless otherwise stated. | |
Record name | Acetyl coenzyme A | |
Source | ChemIDplus | |
URL | https://pubchem.ncbi.nlm.nih.gov/substance/?source=chemidplus&sourceid=0000072899 | |
Description | ChemIDplus is a free, web search system that provides access to the structure and nomenclature authority files used for the identification of chemical substances cited in National Library of Medicine (NLM) databases, including the TOXNET system. | |
Record name | Acetyl CoA | |
Source | EPA DSSTox | |
URL | https://comptox.epa.gov/dashboard/DTXSID30992686 | |
Description | DSSTox provides a high quality public chemistry resource for supporting improved predictive toxicology. | |
Record name | S-acetylcoenzyme A | |
Source | European Chemicals Agency (ECHA) | |
URL | https://echa.europa.eu/substance-information/-/substanceinfo/100.000.719 | |
Description | The European Chemicals Agency (ECHA) is an agency of the European Union which is the driving force among regulatory authorities in implementing the EU's groundbreaking chemicals legislation for the benefit of human health and the environment as well as for innovation and competitiveness. | |
Explanation | Use of the information, documents and data from the ECHA website is subject to the terms and conditions of this Legal Notice, and subject to other binding limitations provided for under applicable law, the information, documents and data made available on the ECHA website may be reproduced, distributed and/or used, totally or in part, for non-commercial purposes provided that ECHA is acknowledged as the source: "Source: European Chemicals Agency, http://echa.europa.eu/". Such acknowledgement must be included in each copy of the material. ECHA permits and encourages organisations and individuals to create links to the ECHA website under the following cumulative conditions: Links can only be made to webpages that provide a link to the Legal Notice page. | |
Record name | ACETYL COENZYME A | |
Source | FDA Global Substance Registration System (GSRS) | |
URL | https://gsrs.ncats.nih.gov/ginas/app/beta/substances/76Q83YLO3O | |
Description | The FDA Global Substance Registration System (GSRS) enables the efficient and accurate exchange of information on what substances are in regulated products. Instead of relying on names, which vary across regulatory domains, countries, and regions, the GSRS knowledge base makes it possible for substances to be defined by standardized, scientific descriptions. | |
Explanation | Unless otherwise noted, the contents of the FDA website (www.fda.gov), both text and graphics, are not copyrighted. They are in the public domain and may be republished, reprinted and otherwise used freely by anyone without the need to obtain permission from FDA. Credit to the U.S. Food and Drug Administration as the source is appreciated but not required. | |
Record name | Acetyl-CoA | |
Source | Human Metabolome Database (HMDB) | |
URL | http://www.hmdb.ca/metabolites/HMDB0001206 | |
Description | The Human Metabolome Database (HMDB) is a freely available electronic database containing detailed information about small molecule metabolites found in the human body. | |
Explanation | HMDB is offered to the public as a freely available resource. Use and re-distribution of the data, in whole or in part, for commercial purposes requires explicit permission of the authors and explicit acknowledgment of the source material (HMDB) and the original publication (see the HMDB citing page). We ask that users who download significant portions of the database cite the HMDB paper in any resulting publications. | |
Retrosynthesis Analysis
AI-Powered Synthesis Planning: Our tool employs the Template_relevance Pistachio, Template_relevance Bkms_metabolic, Template_relevance Pistachio_ringbreaker, Template_relevance Reaxys, Template_relevance Reaxys_biocatalysis model, leveraging a vast database of chemical reactions to predict feasible synthetic routes.
One-Step Synthesis Focus: Specifically designed for one-step synthesis, it provides concise and direct routes for your target compounds, streamlining the synthesis process.
Accurate Predictions: Utilizing the extensive PISTACHIO, BKMS_METABOLIC, PISTACHIO_RINGBREAKER, REAXYS, REAXYS_BIOCATALYSIS database, our tool offers high-accuracy predictions, reflecting the latest in chemical research and data.
Strategy Settings
Precursor scoring | Relevance Heuristic |
---|---|
Min. plausibility | 0.01 |
Model | Template_relevance |
Template Set | Pistachio/Bkms_metabolic/Pistachio_ringbreaker/Reaxys/Reaxys_biocatalysis |
Top-N result to add to graph | 6 |
Feasible Synthetic Routes
体外研究产品的免责声明和信息
请注意,BenchChem 上展示的所有文章和产品信息仅供信息参考。 BenchChem 上可购买的产品专为体外研究设计,这些研究在生物体外进行。体外研究,源自拉丁语 "in glass",涉及在受控实验室环境中使用细胞或组织进行的实验。重要的是要注意,这些产品没有被归类为药物或药品,他们没有得到 FDA 的批准,用于预防、治疗或治愈任何医疗状况、疾病或疾病。我们必须强调,将这些产品以任何形式引入人类或动物的身体都是法律严格禁止的。遵守这些指南对确保研究和实验的法律和道德标准的符合性至关重要。