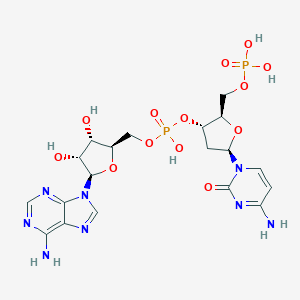
pdCpA
- 点击 快速询问 获取最新报价。
- 提供有竞争力价格的高质量产品,您可以更专注于研究。
描述
PdCpA, or 2'(3')-O-(N-cyclopropylcarboxamide) adenosine-5'-triphosphate, is a modified nucleotide that has gained attention in recent years due to its potential applications in scientific research. The modification of the nucleotide structure allows for unique properties that can be utilized in a variety of research fields.
作用机制
PdCpA acts as an ATP analog, which allows it to interact with enzymes that utilize ATP as a substrate. The cyclopropylcarboxamide group provides unique properties that can alter the binding affinity and specificity of the enzyme for pdCpA. Additionally, pdCpA can be used as a competitive inhibitor of ATP, which can be useful in studying the role of ATP in various cellular processes.
Biochemical and Physiological Effects:
pdCpA has been shown to have various biochemical and physiological effects. It has been shown to be a potent activator of RNA polymerase, which can increase the rate of transcription. pdCpA has also been shown to inhibit the activity of various kinases and phosphatases, which can alter cellular signaling pathways. Additionally, pdCpA has been shown to have an effect on energy metabolism, which can alter cellular energy levels.
实验室实验的优点和局限性
PdCpA has several advantages for lab experiments. It can be used as a tool to study the mechanism of enzymes that utilize ATP as a substrate. Additionally, pdCpA can be used as a competitive inhibitor of ATP, which can be useful in studying the role of ATP in various cellular processes. However, pdCpA has limitations in terms of its stability and solubility. It can degrade over time and can be difficult to dissolve in certain solvents.
未来方向
There are several future directions for the use of pdCpA in scientific research. One potential application is the development of pdCpA-based sensors for ATP detection. Additionally, pdCpA can be used as a tool to study the role of ATP in various cellular processes, including metabolism, signaling, and gene expression. Furthermore, pdCpA can be used as a substrate for the development of new enzymes with altered substrate specificity and binding affinity.
合成方法
The synthesis of pdCpA involves several steps, including the preparation of the starting materials, protection of the hydroxyl groups, and coupling of the cyclopropylcarboxamide group. The final product is obtained through deprotection and purification processes. The synthesis method has been optimized to produce high yields of pdCpA with high purity.
科学研究应用
PdCpA has been utilized in various scientific research fields, including biochemistry, molecular biology, and pharmacology. It has been used to study the mechanism of RNA polymerase, RNA transcription, and translation. pdCpA has also been used as a tool to study the role of ATP in cellular metabolism and energy transfer. Additionally, pdCpA has been used as a substrate for various enzymes, including kinases and phosphatases.
属性
CAS 编号 |
127067-28-1 |
---|---|
产品名称 |
pdCpA |
分子式 |
C19H26N8O13P2 |
分子量 |
636.4 g/mol |
IUPAC 名称 |
[(2R,3S,5R)-5-(4-amino-2-oxopyrimidin-1-yl)-2-(phosphonooxymethyl)oxolan-3-yl] [(2R,3S,4R,5R)-5-(6-aminopurin-9-yl)-3,4-dihydroxyoxolan-2-yl]methyl hydrogen phosphate |
InChI |
InChI=1S/C19H26N8O13P2/c20-11-1-2-26(19(30)25-11)12-3-8(9(38-12)4-36-41(31,32)33)40-42(34,35)37-5-10-14(28)15(29)18(39-10)27-7-24-13-16(21)22-6-23-17(13)27/h1-2,6-10,12,14-15,18,28-29H,3-5H2,(H,34,35)(H2,20,25,30)(H2,21,22,23)(H2,31,32,33)/t8-,9+,10+,12+,14+,15+,18+/m0/s1 |
InChI 键 |
UUBWXCHLJHRYJT-LNAOLWRRSA-N |
手性 SMILES |
C1[C@@H]([C@H](O[C@H]1N2C=CC(=NC2=O)N)COP(=O)(O)O)OP(=O)(O)OC[C@@H]3[C@H]([C@H]([C@@H](O3)N4C=NC5=C(N=CN=C54)N)O)O |
SMILES |
C1C(C(OC1N2C=CC(=NC2=O)N)COP(=O)(O)O)OP(=O)(O)OCC3C(C(C(O3)N4C=NC5=C(N=CN=C54)N)O)O |
规范 SMILES |
C1C(C(OC1N2C=CC(=NC2=O)N)COP(=O)(O)O)OP(=O)(O)OCC3C(C(C(O3)N4C=NC5=C(N=CN=C54)N)O)O |
同义词 |
5'-phospho-2'-deoxyribocytidylylriboadenosine pdCpA |
产品来源 |
United States |
体外研究产品的免责声明和信息
请注意,BenchChem 上展示的所有文章和产品信息仅供信息参考。 BenchChem 上可购买的产品专为体外研究设计,这些研究在生物体外进行。体外研究,源自拉丁语 "in glass",涉及在受控实验室环境中使用细胞或组织进行的实验。重要的是要注意,这些产品没有被归类为药物或药品,他们没有得到 FDA 的批准,用于预防、治疗或治愈任何医疗状况、疾病或疾病。我们必须强调,将这些产品以任何形式引入人类或动物的身体都是法律严格禁止的。遵守这些指南对确保研究和实验的法律和道德标准的符合性至关重要。