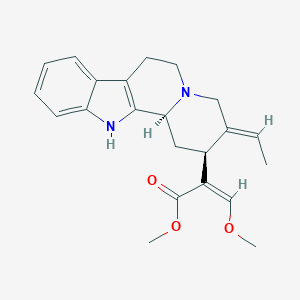
Geissoschizine methyl ether
描述
科学研究应用
Neuroprotective Effects
GM has been studied for its neuroprotective properties against oxidative stress, which is a key factor in neurodegenerative diseases. Research indicates that GM protects neurons from glutamate-induced oxidative cytotoxicity by regulating mitochondrial function and suppressing reactive oxygen species (ROS) generation. This mechanism suggests its potential utility in conditions such as Alzheimer's disease and other forms of dementia.
- Case Study : A study using mouse hippocampal neuronal cell lines demonstrated that GM effectively reduced oxidative stress markers and improved cell viability under glutamate exposure conditions .
Antidepressant Properties
As an active component of the traditional Japanese herbal medicine Yokukansan, GM has been linked to improvements in behavioral and psychological symptoms of dementia (BPSD). Its interaction with serotonin receptors is particularly noteworthy, as it exhibits mixed agonist/antagonist activities on various serotonin receptor subtypes.
- Pharmacological Insights : GM shows significant binding affinity to 5-HT1A and 5-HT2A receptors, indicating its role in modulating serotonergic activity, which is crucial for mood regulation .
Antiepileptic Activity
Recent studies have identified GM as a promising candidate for epilepsy treatment due to its ability to inhibit multiple neuronal channels involved in seizure activity. In vivo experiments demonstrated that GM effectively reduced seizure frequency in various animal models.
- Case Study : In a maximal electroshock-induced seizure model, GM administration resulted in a significant reduction of generalized tonic-clonic seizures at doses ranging from 50 to 100 mg/kg .
Antimicrobial Properties
Emerging research has also explored the antimicrobial potential of GM. Studies have indicated that extracts containing GM exhibit antibacterial activity against drug-resistant strains of bacteria, suggesting its potential role as an antimicrobial agent.
- Research Findings : The antibacterial efficacy of extracts from Illicium verum, which contains GM, was shown to be effective against clinical isolates such as Methicillin-resistant Staphylococcus aureus (MRSA) and Pseudomonas aeruginosa .
Pharmacokinetics and Brain Distribution
Understanding the pharmacokinetics of GM is essential for elucidating its therapeutic potential. Studies have shown that GM can cross the blood-brain barrier, allowing it to exert effects directly within the central nervous system.
- Research Insights : A study utilizing mass spectrometry techniques demonstrated the brain distribution of GM following intravenous administration in rats, confirming its presence in key brain regions associated with cognitive function .
Summary Table: Applications of this compound
准备方法
合成路线和反应条件: 矶松碱甲醚通常是从钩藤中分离出来的 . 分离过程涉及提取和纯化技术,将该化合物从植物中存在的其他生物碱中分离出来。实验室合成方面的具体合成路线和反应条件尚未得到广泛记录,但从天然来源中分离出来仍然是获得该化合物的主要方法。
工业生产方法: 矶松碱甲醚的工业生产涉及从钩藤中进行大规模提取。该过程包括收获植物、干燥,然后使用溶剂提取生物碱。 然后使用色谱技术对提取物进行纯化,以分离矶松碱甲醚 .
化学反应分析
反应类型: 矶松碱甲醚经历各种化学反应,包括氧化、还原和取代 .
常用试剂和条件:
氧化: 可以使用高锰酸钾或过氧化氢等常见的氧化剂。
还原: 通常使用氢化铝锂或硼氢化钠等还原剂。
取代: 亲核取代反应可以与烷基卤化物等试剂发生。
主要产物: 从这些反应中形成的主要产物取决于所使用的具体条件和试剂。 例如,氧化会导致形成矶松碱甲醚 N-氧化物 .
作用机制
矶松碱甲醚主要通过其与血清素受体的相互作用来发挥其作用。 它充当 5-HT7 受体的拮抗剂和 5-HT1A 受体的激动剂 . 这种双重作用调节神经递质释放和神经元兴奋性,有助于其精神活性和抗癫痫作用 .
相似化合物的比较
矶松碱甲醚在吲哚生物碱中是独一无二的,因为它具有特定的受体相互作用。类似的化合物包括:
- 毛茛碱
- 毛茛碱
- 钩藤碱
- 异钩藤碱
- 角萼碱
- 异角萼碱
这些化合物在结构上具有相似性,但在受体结合亲和力和药理作用方面有所不同。 矶松碱甲醚特有的 5-HT7 拮抗作用和 5-HT1A 激动作用使其在治疗潜力方面独树一帜 .
生物活性
Geissoschizine methyl ether (GM) is an alkaloid primarily derived from the Uncaria species, particularly Uncaria rhynchophylla and Uncaria sinensis. It has garnered attention for its diverse pharmacological effects, particularly in the context of neurological and psychological health. This article provides a comprehensive overview of the biological activities associated with GM, including its mechanisms of action, pharmacokinetics, and therapeutic potential.
Serotonergic and Dopaminergic Activity
GM exhibits significant interactions with serotonin receptors, specifically acting as a mixed agonist/antagonist. In vitro studies demonstrate that GM inhibits serotonin receptor binding with varying affinities:
- 5-HT1A receptor : Agonist activity
- 5-HT2A/2C receptors : Antagonist activity
In vivo experiments have shown that GM can reduce head twitch responses induced by 5-hydroxy-L-tryptophan (5-HTP), indicating its potential role in modulating serotonergic pathways. Additionally, GM has been observed to decrease rectal temperature in mice, suggesting hypothermic effects linked to its serotonergic activity .
Neuroprotective Effects
Research indicates that GM plays a role in neuroprotection, particularly in the context of behavioral and psychological symptoms of dementia (BPSD). It is a key component of the traditional Japanese Kampo medicine yokukansan, which is used to ameliorate symptoms such as aggressiveness and irritability. Studies have shown that GM can improve cognitive functions and reduce anxiety-like behaviors in animal models .
Blood-Brain Barrier Permeability
One crucial aspect of GM's efficacy is its ability to cross the blood-brain barrier (BBB). In studies involving oral administration of yokukansan, GM was detected in both plasma and brain tissues, confirming its BBB permeability. This property is essential for its neuroactive effects .
Metabolic Profiling
Metabolic studies have identified several metabolites of GM, primarily hydroxylated forms (HM-1/2), which are generated through cytochrome P450-mediated processes in liver microsomes. The metabolic pathways suggest that GM undergoes significant biotransformation, impacting its pharmacological profile .
Clinical Applications
- Yokukansan and BPSD : Clinical studies have demonstrated that patients treated with yokukansan exhibit marked improvements in BPSD symptoms. The efficacy is attributed largely to GM's pharmacological actions on serotonin and dopamine systems .
- Animal Models : In rodent models, GM administration has resulted in reduced anxiety-like behaviors and improved cognitive functions, supporting its potential use as a therapeutic agent for neurodegenerative conditions .
Summary of Biological Activities
属性
IUPAC Name |
methyl (Z)-2-[(2S,3E,12bS)-3-ethylidene-2,4,6,7,12,12b-hexahydro-1H-indolo[2,3-a]quinolizin-2-yl]-3-methoxyprop-2-enoate | |
---|---|---|
Source | PubChem | |
URL | https://pubchem.ncbi.nlm.nih.gov | |
Description | Data deposited in or computed by PubChem | |
InChI |
InChI=1S/C22H26N2O3/c1-4-14-12-24-10-9-16-15-7-5-6-8-19(15)23-21(16)20(24)11-17(14)18(13-26-2)22(25)27-3/h4-8,13,17,20,23H,9-12H2,1-3H3/b14-4-,18-13-/t17-,20-/m0/s1 | |
Source | PubChem | |
URL | https://pubchem.ncbi.nlm.nih.gov | |
Description | Data deposited in or computed by PubChem | |
InChI Key |
VAMJZLUOKJRHOW-XEASWFAXSA-N | |
Source | PubChem | |
URL | https://pubchem.ncbi.nlm.nih.gov | |
Description | Data deposited in or computed by PubChem | |
Canonical SMILES |
CC=C1CN2CCC3=C(C2CC1C(=COC)C(=O)OC)NC4=CC=CC=C34 | |
Source | PubChem | |
URL | https://pubchem.ncbi.nlm.nih.gov | |
Description | Data deposited in or computed by PubChem | |
Isomeric SMILES |
C/C=C\1/CN2CCC3=C([C@@H]2C[C@@H]1/C(=C/OC)/C(=O)OC)NC4=CC=CC=C34 | |
Source | PubChem | |
URL | https://pubchem.ncbi.nlm.nih.gov | |
Description | Data deposited in or computed by PubChem | |
Molecular Formula |
C22H26N2O3 | |
Source | PubChem | |
URL | https://pubchem.ncbi.nlm.nih.gov | |
Description | Data deposited in or computed by PubChem | |
DSSTOX Substance ID |
DTXSID101045604 | |
Record name | Geissoschizine methyl ether | |
Source | EPA DSSTox | |
URL | https://comptox.epa.gov/dashboard/DTXSID101045604 | |
Description | DSSTox provides a high quality public chemistry resource for supporting improved predictive toxicology. | |
Molecular Weight |
366.5 g/mol | |
Source | PubChem | |
URL | https://pubchem.ncbi.nlm.nih.gov | |
Description | Data deposited in or computed by PubChem | |
CAS No. |
60314-89-8 | |
Record name | Geissoschizine methyl ether | |
Source | CAS Common Chemistry | |
URL | https://commonchemistry.cas.org/detail?cas_rn=60314-89-8 | |
Description | CAS Common Chemistry is an open community resource for accessing chemical information. Nearly 500,000 chemical substances from CAS REGISTRY cover areas of community interest, including common and frequently regulated chemicals, and those relevant to high school and undergraduate chemistry classes. This chemical information, curated by our expert scientists, is provided in alignment with our mission as a division of the American Chemical Society. | |
Explanation | The data from CAS Common Chemistry is provided under a CC-BY-NC 4.0 license, unless otherwise stated. | |
Record name | Geissoschizine methyl ether | |
Source | ChemIDplus | |
URL | https://pubchem.ncbi.nlm.nih.gov/substance/?source=chemidplus&sourceid=0060314898 | |
Description | ChemIDplus is a free, web search system that provides access to the structure and nomenclature authority files used for the identification of chemical substances cited in National Library of Medicine (NLM) databases, including the TOXNET system. | |
Record name | Geissoschizine methyl ether | |
Source | EPA DSSTox | |
URL | https://comptox.epa.gov/dashboard/DTXSID101045604 | |
Description | DSSTox provides a high quality public chemistry resource for supporting improved predictive toxicology. | |
Record name | GEISSOSCHIZINE METHYL ETHER | |
Source | FDA Global Substance Registration System (GSRS) | |
URL | https://gsrs.ncats.nih.gov/ginas/app/beta/substances/TNN2THT2NX | |
Description | The FDA Global Substance Registration System (GSRS) enables the efficient and accurate exchange of information on what substances are in regulated products. Instead of relying on names, which vary across regulatory domains, countries, and regions, the GSRS knowledge base makes it possible for substances to be defined by standardized, scientific descriptions. | |
Explanation | Unless otherwise noted, the contents of the FDA website (www.fda.gov), both text and graphics, are not copyrighted. They are in the public domain and may be republished, reprinted and otherwise used freely by anyone without the need to obtain permission from FDA. Credit to the U.S. Food and Drug Administration as the source is appreciated but not required. | |
Retrosynthesis Analysis
AI-Powered Synthesis Planning: Our tool employs the Template_relevance Pistachio, Template_relevance Bkms_metabolic, Template_relevance Pistachio_ringbreaker, Template_relevance Reaxys, Template_relevance Reaxys_biocatalysis model, leveraging a vast database of chemical reactions to predict feasible synthetic routes.
One-Step Synthesis Focus: Specifically designed for one-step synthesis, it provides concise and direct routes for your target compounds, streamlining the synthesis process.
Accurate Predictions: Utilizing the extensive PISTACHIO, BKMS_METABOLIC, PISTACHIO_RINGBREAKER, REAXYS, REAXYS_BIOCATALYSIS database, our tool offers high-accuracy predictions, reflecting the latest in chemical research and data.
Strategy Settings
Precursor scoring | Relevance Heuristic |
---|---|
Min. plausibility | 0.01 |
Model | Template_relevance |
Template Set | Pistachio/Bkms_metabolic/Pistachio_ringbreaker/Reaxys/Reaxys_biocatalysis |
Top-N result to add to graph | 6 |
Feasible Synthetic Routes
Q1: What are the primary pharmacological targets of GM?
A1: GM interacts with multiple targets in the central nervous system, exhibiting affinity for various serotonin (5-HT) receptor subtypes, including 5-HT1A, 5-HT1B, 5-HT2A, 5-HT2B, 5-HT2C, 5-HT4, 5-HT5A, 5-HT6, and 5-HT7. [, , ] It demonstrates partial agonistic activity at 5-HT1A receptors and antagonistic activity at 5-HT2A, 5-HT2B, 5-HT2C, and 5-HT7 receptors. [] Additionally, GM exhibits acetylcholinesterase (AChE) inhibitory activity. [, , ]
Q2: How does GM's interaction with 5-HT receptors contribute to its potential therapeutic effects?
A2: GM's partial agonism at 5-HT1A receptors is linked to its anti-aggressive effects, [, ] while its antagonism at 5-HT2A receptors might contribute to its potential antipsychotic-like activity. [, ] The modulation of multiple 5-HT receptor subtypes suggests a complex interplay that contributes to its diverse pharmacological profile.
Q3: Does GM affect other neurotransmitter systems?
A3: Research suggests that GM may influence the dopaminergic system by inhibiting catechol-O-methyltransferase (COMT), an enzyme involved in dopamine metabolism. [] This inhibition could lead to increased dopamine levels, potentially contributing to some of GM's effects.
Q4: What are the downstream effects of GM's AChE inhibitory activity?
A4: By inhibiting AChE, GM can increase acetylcholine levels in the brain. [, , ] This mechanism is particularly relevant to its potential in managing conditions like Alzheimer's disease, where acetylcholine deficiency is a hallmark.
Q5: How does GM contribute to the neuroprotective effects of Uncaria hook?
A5: Studies indicate that GM, alongside other alkaloids in Uncaria hook, might exert neuroprotective effects through various mechanisms, including anti-oxidant, anti-inflammatory, and neuromodulatory activities. [, , ] These properties are being investigated for their potential in neurodegenerative diseases.
Q6: What is known about the absorption and distribution of GM?
A6: GM is absorbed into the bloodstream following oral administration of Uncaria hook preparations. [, , ] Importantly, it can cross the blood-brain barrier, reaching the brain, which is crucial for its central nervous system activity. [, , ]
Q7: How is GM metabolized in the body?
A7: GM is primarily metabolized by cytochrome P450 (CYP) enzymes, mainly CYP3A4, into various metabolites. [, , ] The metabolic profile includes oxidized, demethylated, and water-adduct forms. [, ] Notably, there might be gender-related differences in GM metabolism in rats, although not observed in humans. [, ]
Q8: What are the routes of GM elimination?
A8: Following metabolism, GM and its metabolites are excreted through various routes, including urine and bile. [, ] The specific contributions of each route may vary depending on factors such as dosage and individual variations.
Q9: What in vitro models have been used to study the effects of GM?
A9: Various cell-based assays have been employed to investigate GM's effects, including primary cultured rat cortical neurons, [] PC12 cells (rat pheochromocytoma cells), [] and HT22 cells (mouse hippocampal neuronal cells). [] These models have provided insights into its neuroprotective, anti-inflammatory, and neurotrophic properties.
Q10: What in vivo models have been used to study the effects of GM?
A10: Rodent models, particularly rats and mice, have been extensively used to study the effects of GM. These include models of Alzheimer's disease, [] Parkinson's disease, [] depression, [] and behavioral disorders. [, ]
Q11: Are there any clinical trials investigating the therapeutic potential of GM?
A11: While clinical trials have assessed the efficacy of Uncaria hook preparations containing GM, specifically yokukansan, for conditions like behavioral and psychological symptoms of dementia (BPSD), [, , ] dedicated clinical trials focusing solely on GM are limited.
Q12: What is the molecular formula and weight of GM?
A12: GM has a molecular formula of C22H28N2O4 and a molecular weight of 384.47 g/mol. []
Q13: What spectroscopic data is available for the characterization of GM?
A13: GM's structure has been elucidated using various spectroscopic techniques, including ultraviolet-visible (UV-Vis) spectroscopy, electrospray ionization quadrupole time-of-flight mass spectrometry (ESI-QTOF MS), and nuclear magnetic resonance (NMR) spectroscopy. [, , , ] These techniques provide detailed information about its functional groups, molecular weight, and three-dimensional structure.
体外研究产品的免责声明和信息
请注意,BenchChem 上展示的所有文章和产品信息仅供信息参考。 BenchChem 上可购买的产品专为体外研究设计,这些研究在生物体外进行。体外研究,源自拉丁语 "in glass",涉及在受控实验室环境中使用细胞或组织进行的实验。重要的是要注意,这些产品没有被归类为药物或药品,他们没有得到 FDA 的批准,用于预防、治疗或治愈任何医疗状况、疾病或疾病。我们必须强调,将这些产品以任何形式引入人类或动物的身体都是法律严格禁止的。遵守这些指南对确保研究和实验的法律和道德标准的符合性至关重要。