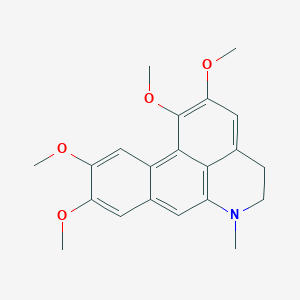
脱氢格劳辛
描述
Dehydroglaucine is a compound with the CAS Number: 22212-26-6 and a Molecular Weight of 353.42 . It is also known as 1,2,9,10-tetramethoxy-6-methyl-5,6-dihydro-4H-dibenzo [de,g]quinoline .
Synthesis Analysis
The synthesis of Dehydroglaucine involves the dehydrogenation of glaucine by mercury acetate . The process utilizes the tendency of cyclic tertiary amines to dehydrogenate and form a C=C double bond in the a,b-position to the N atom . Investigations showed that mercury acetate in the presence of NaBH4 dehydrogenates glaucine regioselectively to form 6,7-dehydroglaucine .Molecular Structure Analysis
The structure of Dehydroglaucine was confirmed by IR, PMR, and 13C NMR spectra and an x-ray structure analysis . The bond lengths and angles are close to the usual values except for the C6=C7 double bond at 1.355 Å .Chemical Reactions Analysis
Unfortunately, I could not find specific information on the chemical reactions involving Dehydroglaucine .Physical And Chemical Properties Analysis
Dehydroglaucine has a molecular formula of C21H23NO4 and a molecular weight of 353.41 . It is recommended to be stored at -20°C for 3 years or at 4°C for 2 years .科学研究应用
- 6,7-Dehydroglaucine has been investigated for its potential antidepressant effects. Researchers have explored its interaction with the sodium-dependent serotonin transporter, a key target for antidepressant drugs .
- The structure of 6,7-Dehydroglaucine has been confirmed through IR, PMR, and 13C NMR spectra, as well as x-ray structure analysis .
Antidepressant Activity
Chemical Synthesis and Structure
Alkaloid Derivatives
作用机制
Target of Action
Dehydroglaucine is a potent antimicrobial alkaloid . It has been found to exhibit inhibitory activity against a variety of microorganisms, including Staphylococcus aureus, Mycobacterium smegmatis, Candida albicans, and Aspergillus niger .
Result of Action
The primary result of Dehydroglaucine’s action is its antimicrobial effect . By inhibiting the growth of or killing microorganisms, Dehydroglaucine can help to control infections caused by these organisms .
未来方向
属性
IUPAC Name |
4,5,15,16-tetramethoxy-10-methyl-10-azatetracyclo[7.7.1.02,7.013,17]heptadeca-1,3,5,7,9(17),13,15-heptaene | |
---|---|---|
Source | PubChem | |
URL | https://pubchem.ncbi.nlm.nih.gov | |
Description | Data deposited in or computed by PubChem | |
InChI |
InChI=1S/C21H23NO4/c1-22-7-6-12-9-18(25-4)21(26-5)20-14-11-17(24-3)16(23-2)10-13(14)8-15(22)19(12)20/h8-11H,6-7H2,1-5H3 | |
Source | PubChem | |
URL | https://pubchem.ncbi.nlm.nih.gov | |
Description | Data deposited in or computed by PubChem | |
InChI Key |
RZUHGAKUNBFQJS-UHFFFAOYSA-N | |
Source | PubChem | |
URL | https://pubchem.ncbi.nlm.nih.gov | |
Description | Data deposited in or computed by PubChem | |
Canonical SMILES |
CN1CCC2=CC(=C(C3=C4C=C(C(=CC4=CC1=C23)OC)OC)OC)OC | |
Source | PubChem | |
URL | https://pubchem.ncbi.nlm.nih.gov | |
Description | Data deposited in or computed by PubChem | |
Molecular Formula |
C21H23NO4 | |
Source | PubChem | |
URL | https://pubchem.ncbi.nlm.nih.gov | |
Description | Data deposited in or computed by PubChem | |
DSSTOX Substance ID |
DTXSID30176762 | |
Record name | 4H-Dibenzo(de,g)quinoline, 5,6-dihydro-1,2,9,10-tetramethoxy-6-methyl- | |
Source | EPA DSSTox | |
URL | https://comptox.epa.gov/dashboard/DTXSID30176762 | |
Description | DSSTox provides a high quality public chemistry resource for supporting improved predictive toxicology. | |
Molecular Weight |
353.4 g/mol | |
Source | PubChem | |
URL | https://pubchem.ncbi.nlm.nih.gov | |
Description | Data deposited in or computed by PubChem | |
Product Name |
Dehydroglaucine | |
CAS RN |
22212-26-6 | |
Record name | Didehydroglaucine | |
Source | ChemIDplus | |
URL | https://pubchem.ncbi.nlm.nih.gov/substance/?source=chemidplus&sourceid=0022212266 | |
Description | ChemIDplus is a free, web search system that provides access to the structure and nomenclature authority files used for the identification of chemical substances cited in National Library of Medicine (NLM) databases, including the TOXNET system. | |
Record name | 4H-Dibenzo(de,g)quinoline, 5,6-dihydro-1,2,9,10-tetramethoxy-6-methyl- | |
Source | EPA DSSTox | |
URL | https://comptox.epa.gov/dashboard/DTXSID30176762 | |
Description | DSSTox provides a high quality public chemistry resource for supporting improved predictive toxicology. | |
Retrosynthesis Analysis
AI-Powered Synthesis Planning: Our tool employs the Template_relevance Pistachio, Template_relevance Bkms_metabolic, Template_relevance Pistachio_ringbreaker, Template_relevance Reaxys, Template_relevance Reaxys_biocatalysis model, leveraging a vast database of chemical reactions to predict feasible synthetic routes.
One-Step Synthesis Focus: Specifically designed for one-step synthesis, it provides concise and direct routes for your target compounds, streamlining the synthesis process.
Accurate Predictions: Utilizing the extensive PISTACHIO, BKMS_METABOLIC, PISTACHIO_RINGBREAKER, REAXYS, REAXYS_BIOCATALYSIS database, our tool offers high-accuracy predictions, reflecting the latest in chemical research and data.
Strategy Settings
Precursor scoring | Relevance Heuristic |
---|---|
Min. plausibility | 0.01 |
Model | Template_relevance |
Template Set | Pistachio/Bkms_metabolic/Pistachio_ringbreaker/Reaxys/Reaxys_biocatalysis |
Top-N result to add to graph | 6 |
Feasible Synthetic Routes
Q & A
Q1: What is dehydroglaucine and where is it found?
A1: Dehydroglaucine is an aporphine alkaloid found in several plant species. It has been isolated from plants belonging to the Papaveraceae family, such as Glaucium flavum [], Corydalis yanhusuo [], and Glaucium aleppicum [], as well as from the heartwood of the Liriodendron tulipifera tree [].
Q2: What are the pharmacological effects of dehydroglaucine and its analogs?
A2: Dehydroglaucine and its structurally related analogs have been studied for various pharmacological activities. Research suggests they may possess hypotensive effects [], antiplatelet aggregation properties [], antimicrobial activity [], and potential as acetylcholinesterase inhibitors []. Some analogs exhibit inhibitory effects on mouse splenocyte activity, impacting both lipopolysaccharide (LPS)-induced and concanavalin A (Con A)-induced proliferation [].
Q3: How does the structure of dehydroglaucine compare to glaucine, and how do these structural differences affect their activity?
A3: Dehydroglaucine is a dehydrogenated analog of glaucine, meaning it possesses a double bond where glaucine has a single bond. This structural modification leads to quantitative and qualitative changes in pharmacological activity. For example, dehydroglaucine demonstrates a more gradual and pronounced hypotensive effect compared to the sharp, initial drop caused by glaucine []. Additionally, while glaucine shows antitussive activity, 7-methyl dehydroglaucine is the only analog found to share this property [].
Q4: Can you elaborate on the hypotensive effects of dehydroglaucine and its interaction with neurotransmitters?
A4: Dehydroglaucine and its analogs induce a slow-onset, prolonged decrease in blood pressure []. Interestingly, unlike glaucine, they don't significantly impact respiration or cardiac activity in experimental animals []. Dehydroglaucine has been shown to modulate the effects of neurotransmitters like noradrenaline and nicotine. While glaucine generally suppresses their pressor effects, the impact of dehydroglaucine is dose-dependent, ranging from moderate suppression to complete inhibition [].
Q5: What is known about the antiplatelet aggregation activity of dehydroglaucine?
A5: Dehydroglaucine, along with other oxoaporphine and 6a,7-dehydroaporphine alkaloids isolated from Annona purpurea, exhibited significant antiplatelet aggregation activity []. This suggests a potential therapeutic application for dehydroglaucine in cardiovascular diseases.
Q6: How does dehydroglaucine exert its antimicrobial effects?
A6: Dehydroglaucine, isolated from the heartwood of Liriodendron tulipifera, displayed antimicrobial activity against Staphylococcus aureus, Mycobacterium smegmatis, Candida albicans, and Aspergillus niger []. The specific mechanism of action against these microorganisms remains to be fully elucidated.
Q7: What is the significance of dehydroglaucine being identified as an acetylcholinesterase inhibitor?
A7: The identification of dehydroglaucine as an acetylcholinesterase inhibitor from Corydalis yanhusuo [] highlights its potential therapeutic value for neurodegenerative diseases like Alzheimer's disease, where acetylcholinesterase inhibition is a key therapeutic target.
Q8: What are the implications of the diverse alkaloid profiles found in different populations of Glaucium flavum?
A8: The variation in isoquinoline alkaloid profiles observed in geographically distinct populations of Glaucium flavum [] highlights the potential influence of environmental factors on alkaloid biosynthesis. This variability could impact the pharmacological properties of extracts derived from different populations.
Q9: What analytical techniques have been employed to identify and characterize dehydroglaucine in plant extracts?
A9: Various analytical techniques have been utilized to identify and characterize dehydroglaucine in plant extracts. These include gas chromatography-mass spectrometry (GC-MS) [], ultra-high performance liquid chromatography coupled with quadrupole-time-of-flight mass spectrometry (UPLC-Q-TOF-MS) [], and ultra-high performance liquid chromatography-photodiode array-high resolution tandem mass spectrometry (UPLC-PDA-HRMS-MS/MS) [].
Q10: What is the significance of using zeolite-based solid-phase extraction for analyzing dehydroglaucine?
A10: The implementation of a zeolite-based solid-phase extraction method coupled with UPLC-Q-TOF-MS enabled the rapid and efficient extraction and analysis of dehydroglaucine and other acetylcholinesterase inhibitors from Corydalis yanhusuo []. This method provides a valuable tool for identifying bioactive compounds from complex plant matrices.
Q11: Have there been any studies investigating the structure-activity relationship of dehydroglaucine and its analogs?
A11: Yes, research has explored the structure-activity relationship of dehydroglaucine analogs. Modifications to the glaucine structure, such as dehydrogenation and N-oxidation, have been shown to significantly influence toxicity, central nervous system inhibition, antitussive activity, and spasmolytic action []. These findings highlight the importance of structural modifications in fine-tuning the pharmacological profiles of these alkaloids.
Q12: What is the significance of identifying new alkaloids like yuanhunine from Corydalis turtschaninovii?
A12: The discovery of new alkaloids, like yuanhunine from Corydalis turtschaninovii [], expands the chemical diversity of natural products and opens avenues for exploring novel pharmacological activities. This highlights the ongoing potential of natural product research in drug discovery.
Q13: What are the potential benefits of studying the quinone reductase inducing activities of alkaloids like dehydroglaucine?
A13: Investigating the quinone reductase inducing activities of alkaloids like dehydroglaucine from Corydalis Rhizoma [] is crucial for understanding their potential chemopreventive properties. Quinone reductase is a key enzyme involved in detoxification and its induction is associated with a reduced risk of cancer development.
Q14: What are the implications of isolating saulatine from Corydalis yanhusuo for the first time?
A14: The isolation of saulatine, an isoquinobenzazepine alkaloid, from Corydalis yanhusuo for the first time [] broadens our understanding of the phytochemical diversity within this species. This finding further emphasizes the potential of this plant as a source of bioactive compounds.
Q15: What is the significance of characterizing the chemical constituents of Dactylicapnos scandens?
A15: The characterization of eleven alkaloids, including dehydroglaucine, from Dactylicapnos scandens [] contributes to the chemotaxonomic knowledge of this plant species. Additionally, it provides a basis for further research into the potential pharmacological activities of these isolated compounds.
Q16: What are the implications of identifying dehydroglaucine in Glaucium aleppicum for the first time?
A16: The identification of dehydroglaucine in Glaucium aleppicum for the first time, along with other alkaloids [], expands the known chemical profile of this species. This finding contributes to a better understanding of the chemotaxonomic relationships within the genus Glaucium.
体外研究产品的免责声明和信息
请注意,BenchChem 上展示的所有文章和产品信息仅供信息参考。 BenchChem 上可购买的产品专为体外研究设计,这些研究在生物体外进行。体外研究,源自拉丁语 "in glass",涉及在受控实验室环境中使用细胞或组织进行的实验。重要的是要注意,这些产品没有被归类为药物或药品,他们没有得到 FDA 的批准,用于预防、治疗或治愈任何医疗状况、疾病或疾病。我们必须强调,将这些产品以任何形式引入人类或动物的身体都是法律严格禁止的。遵守这些指南对确保研究和实验的法律和道德标准的符合性至关重要。