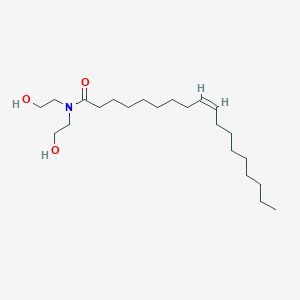
Oleic diethanolamide
描述
Oleic diethanolamide (ODEA), also known as oleamide DEA (CAS No. 93-83-4), is a nonionic surfactant synthesized via the reaction of oleic acid (a monounsaturated C18:1 fatty acid) with diethanolamine . Its molecular formula is C₂₂H₄₃NO₃, and it exhibits excellent emulsifying, foaming, and antistatic properties. ODEA is widely utilized in personal care products (e.g., shampoos, conditioners), industrial cleaners, metalworking fluids, and as a nematicidal agent . It is biodegradable and operates effectively in both polar and nonpolar media due to its amphiphilic structure .
Key synthesis parameters include a 1:3 molar ratio of oleic acid to diethanolamine, reaction temperatures of 70–170°C, and catalyst use (e.g., K₂CO₃), achieving 61–78% conversion rates depending on solvent conditions .
作用机制
Target of Action
Oleic diethanolamide (Oleic DEA) is a non-ionic surfactant It’s structurally similar compound, ethanolamine oleate, has been reported to interact with calcium ions and coagulation factor xii .
Mode of Action
The oleic acid component of ethanolamine oleate, a compound similar to Oleic DEA, is responsible for the inflammatory response and may also activate coagulation in vivo by release of tissue factor and activation of Hageman factor
Biochemical Pathways
A related compound, oleoylethanolamide (oea), has been reported to modulate feeding, body weight, and lipid metabolism by binding with high affinity to the ligand-activated transcription factor, peroxisome proliferator-activated receptor-alpha (ppar-α) .
Result of Action
It has been synthesized through amidification of diethanolamine (dea) with oleic acid (oa) using immobile lipase as a biocatalyst . The synthesized Oleic DEA was characterized by Fourier Transform Infra-Red (FTIR) spectroscopy .
Action Environment
Oleic DEA is commonly used as a detergency booster, anticorrosive, and skin-protective agent in industrial degreasing and cleaning agents, such as engine cleaners . It has also been used as a surfactant nanocarrier for enhanced oil recovery processes . The action, efficacy, and stability of Oleic DEA can be influenced by environmental factors such as temperature, pH, and the presence of other substances in the environment.
生化分析
Biochemical Properties
Oleic diethanolamide plays a significant role in biochemical reactions due to its surfactant properties. It interacts with various enzymes, proteins, and other biomolecules, facilitating the formation of micelles and enhancing the solubility of hydrophobic compounds. One notable interaction is with lipase enzymes, which catalyze the hydrolysis of fats. This compound can act as a substrate for lipase, leading to the formation of oleic acid and diethanolamine . Additionally, it interacts with proteins involved in cell membrane stability and signaling, such as phospholipids and cholesterol, by integrating into the lipid bilayer and altering membrane fluidity .
Cellular Effects
This compound influences various cellular processes, including cell signaling pathways, gene expression, and cellular metabolism. It has been shown to modulate the activity of protein kinase C (PKC), a key enzyme in signal transduction pathways . By activating PKC, this compound can influence cell proliferation, differentiation, and apoptosis. Furthermore, it affects gene expression by interacting with transcription factors such as peroxisome proliferator-activated receptor alpha (PPARα), which regulates lipid metabolism and inflammation . This compound also impacts cellular metabolism by enhancing the uptake and utilization of fatty acids, leading to increased energy production .
Molecular Mechanism
The molecular mechanism of this compound involves its interaction with various biomolecules, leading to changes in enzyme activity, gene expression, and cellular function. At the molecular level, this compound binds to and activates PPARα, a nuclear receptor that regulates the expression of genes involved in lipid metabolism . This activation leads to increased fatty acid oxidation and reduced triglyceride levels. Additionally, this compound can inhibit the activity of acetyl-CoA carboxylase (ACC), an enzyme involved in fatty acid synthesis, thereby reducing lipid accumulation . These interactions highlight the role of this compound in regulating lipid homeostasis and energy metabolism.
Temporal Effects in Laboratory Settings
In laboratory settings, the effects of this compound can vary over time due to its stability, degradation, and long-term impact on cellular function. Studies have shown that this compound is relatively stable under physiological conditions, with minimal degradation over time . Prolonged exposure to this compound can lead to changes in cellular function, including alterations in membrane fluidity and enzyme activity . These temporal effects are important considerations when studying the long-term impact of this compound on cellular processes.
Dosage Effects in Animal Models
The effects of this compound vary with different dosages in animal models. At low doses, this compound has been shown to enhance lipid metabolism and reduce inflammation . At high doses, it can exhibit toxic effects, including hepatotoxicity and oxidative stress . These dosage-dependent effects highlight the importance of determining the optimal dosage for therapeutic applications while minimizing potential adverse effects.
Metabolic Pathways
This compound is involved in several metabolic pathways, primarily related to lipid metabolism. It is metabolized by lipase enzymes, leading to the formation of oleic acid and diethanolamine . Oleic acid can then enter the β-oxidation pathway for energy production, while diethanolamine can be further metabolized or excreted . Additionally, this compound can influence metabolic flux by modulating the activity of key enzymes involved in lipid synthesis and degradation .
Transport and Distribution
This compound is transported and distributed within cells and tissues through interactions with transporters and binding proteins. It can be incorporated into lipoproteins, such as low-density lipoprotein (LDL) and high-density lipoprotein (HDL), facilitating its transport in the bloodstream . Within cells, this compound can bind to fatty acid-binding proteins (FABPs), which aid in its intracellular transport and localization . These interactions ensure the efficient distribution of this compound to target tissues and cells.
Subcellular Localization
The subcellular localization of this compound is primarily within the lipid bilayer of cell membranes. It can integrate into the phospholipid bilayer, altering membrane fluidity and affecting membrane-associated processes . Additionally, this compound can be localized to lipid droplets, where it plays a role in lipid storage and metabolism . These subcellular localizations are crucial for the functional activity of this compound in regulating cellular processes.
生物活性
Oleic diethanolamide, also known as oleamide DEA, is a fatty acid amide derived from oleic acid and diethanolamine. This compound has garnered attention due to its diverse biological activities and applications in various fields, including pharmaceuticals, cosmetics, and industrial processes. This article delves into the biological activity of this compound, highlighting its synthesis, mechanisms of action, and relevant case studies.
Synthesis and Characterization
This compound is synthesized through the amidation of oleic acid with diethanolamine. Studies have optimized this synthesis using various methods, including solvent-free systems and enzymatic catalysis with immobilized lipase. For instance, a study reported an optimal conversion rate of 78.01% under specific conditions (enzyme amount, substrate molar ratio, and temperature) using response surface methodology (RSM) .
Characterization Techniques:
- Nuclear Magnetic Resonance (NMR): Characteristic peaks in the NMR spectrum confirm the successful synthesis of this compound .
- Infrared Spectroscopy (FTIR): FTIR analysis indicates the presence of functional groups typical for amides and confirms the elimination of starting amines during synthesis .
This compound exhibits multiple biological activities primarily attributed to its surfactant properties. It acts as a non-ionic surfactant that can influence various biochemical pathways:
- Surface Activity: this compound demonstrates excellent surface-active properties, making it effective in applications such as emulsifiers and corrosion inhibitors .
- Interaction with Calcium Ions: Similar compounds have been shown to interact with calcium ions, potentially influencing coagulation processes .
- Inflammatory Response Modulation: The oleic acid component may activate inflammatory responses by modulating tissue factors involved in coagulation .
Antirust Performance
This compound has been researched for its antirust properties, showing promise as an effective corrosion inhibitor in industrial applications. Its ability to form protective layers on metal surfaces enhances its utility in this domain .
Toxicological Studies
Toxicological evaluations have been conducted to assess the safety profile of this compound. A notable study involved dermal administration in mice over 13 weeks, revealing minimal to moderate skin irritation at higher doses. However, survival rates were comparable to control groups, indicating a relatively safe profile at lower concentrations .
Case Studies
-
Enzymatic Synthesis Optimization:
A study focused on optimizing the enzymatic synthesis of oleoyl-diethanolamide using immobilized lipase. The results indicated that increasing enzyme concentration and reaction time significantly enhanced conversion rates, showcasing the potential for efficient industrial applications . -
Toxicological Assessment:
In a comprehensive toxicology study involving long-term exposure in mice, oleic acid diethanolamine condensate was administered dermally. The study found that while some skin irritation occurred at higher doses, overall survival rates remained stable across treated groups .
Research Findings Summary
Study Focus | Key Findings |
---|---|
Synthesis Optimization | Achieved 78.01% conversion using RSM; optimal conditions identified |
Antirust Performance | Effective as a corrosion inhibitor; forms protective layers on metals |
Toxicological Safety | Minimal skin irritation observed; comparable survival rates to controls |
Mechanism of Action | Modulates inflammatory responses; interacts with calcium ions |
科学研究应用
Surfactant Properties
Oleic diethanolamide exhibits excellent surfactant properties, making it valuable in various formulations:
- Detergents and Cleaners : It is widely used in industrial cleaning and household detergents due to its ability to reduce surface tension and enhance cleaning efficiency .
- Emulsifiers : The compound serves as a nonionic emulsifier in cosmetics and personal care products, stabilizing emulsions and improving product texture .
Lubrication Applications
The compound has been extensively studied for its role as a lubricant additive:
- Tribological Properties : this compound enhances the tribological performance of lubricants by forming protective films on metal surfaces, thus reducing friction and wear .
- Biodegradability : Research indicates that this compound improves the biodegradability of lubricating oils while enhancing their anti-wear properties .
Corrosion Inhibition
This compound functions effectively as a corrosion inhibitor:
- Metal Protection : Studies demonstrate its ability to protect metals from corrosion in aqueous environments, making it suitable for use in various industrial applications .
- Nanocarrier Systems : Recent research has explored using this compound in conjunction with nanocarriers for enhanced corrosion protection .
Nutritional Science
In nutritional research, this compound has shown potential benefits:
- Lipid Metabolism : It is studied for its effects on lipid metabolism and energy intake regulation, indicating potential applications in weight management strategies .
- Food Intake Control : Research suggests that this compound may influence food intake control mechanisms, contributing to obesity treatment approaches .
Enhanced Oil Recovery
This compound is being evaluated for its role in enhanced oil recovery processes:
- Surfactant Efficiency : Studies have shown that the compound can reduce surfactant losses during oil recovery operations, improving overall efficiency .
Biodegradable Lubricants
The development of environmentally friendly lubricants is another significant application area:
- Additive Research : this compound has been investigated as an additive in mineral lubricating oils to enhance biodegradability and lubricity, aligning with sustainability goals in lubricant formulation .
Case Studies and Research Findings
化学反应分析
Reaction Conditions
-
Temperature : The reaction typically occurs at elevated temperatures (around 182 °C) to facilitate the removal of water produced during the reaction.
-
Catalysts : Sodium hydroxide is often used as a catalyst to enhance the reaction rate. Other catalysts may include immobilized lipases, which have been shown to optimize the amidification process in solvent-free systems.
Optimization Studies
Research employing Response Surface Methodology (RSM) has identified key variables influencing the reaction yield, including:
-
Temperature
-
Reaction time
-
Enzyme amount
-
Substrate molar ratio (diethanolamine to oleic acid)
-
Stages of amine addition
These variables have been systematically varied to determine optimal conditions for maximizing oleoyl-diethanolamide yield while minimizing costs .
Characterization of Oleic Diethanolamide
Characterization of this compound is essential for confirming its structure and purity. Techniques used include:
-
Fourier Transform Infrared Spectroscopy (FTIR) : This technique identifies functional groups present in the compound, such as amide bonds (C=O stretching at approximately 1621 cm) and C-N stretching vibrations.
-
Nuclear Magnetic Resonance (NMR) : NMR spectroscopy provides insights into the molecular structure by revealing information about hydrogen environments within the molecule.
FTIR Spectrum Analysis
The FTIR spectrum of this compound typically shows:
-
Peaks corresponding to hydroxyl groups (OH) around 1366 cm
-
Aliphatic C-H stretching vibrations at approximately 2924 cm and 2853 cm
-
Amide C=O stretching at around 1621 cm and C-N stretching at approximately 1068 cm
Chemical Stability and Decomposition
This compound exhibits stability under normal conditions but can decompose under extreme heat or highly acidic/basic environments. The decomposition products include oleic acid and diethanolamine, which can be significant when considering its application in formulations that may experience varying pH levels or temperatures.
Decomposition Reaction
The decomposition can be represented as follows:
Biological Activities
Research indicates that this compound possesses various biological activities:
-
Antimicrobial Properties : Studies suggest that it may exhibit antimicrobial effects, making it useful in personal care products.
-
Skin Penetration Enhancement : Its nonionic nature allows for favorable interactions with biological membranes, enhancing skin penetration in topical formulations.
常见问题
Q. Basic: What are the standard synthesis methods for oleic diethanolamide, and how do reaction parameters influence yield and purity?
Answer:
this compound is typically synthesized via a condensation reaction between oleic acid and diethanolamine (DEA). Key parameters include:
- Molar ratio : A 1:1 molar ratio of oleic acid to DEA is common, though excess DEA may reduce free amine content .
- Temperature : Optimal reaction temperatures range from 160–182°C, with prolonged heating (2–3 hours) to remove water .
- Catalysts : Alkaline catalysts (e.g., K₂CO₃-modified zeolites) enhance amidation efficiency .
- Enzymatic synthesis : Lipases under solvent-free systems achieve ~61% conversion at 70°C, with DEA acting as a solvent .
Data Table :
Method | Temperature (°C) | Time (h) | Yield/Purity | Key Reference |
---|---|---|---|---|
Conventional amidation | 182 | 2 | High purity, low DEA | |
Enzymatic (solvent-free) | 70 | 24 | 61.35% conversion |
Q. Basic: Which analytical techniques are routinely used to characterize this compound and its derivatives?
Answer:
Common techniques include:
- Chromatography : HPLC and GC-MS separate epoxidized/non-epoxidized diethanolamides and monitor reaction progress .
- Spectroscopy : FTIR confirms amide bond formation (e.g., C=O stretch at ~1640 cm⁻¹) .
- Surface tension measurements : Evaluate surfactant efficacy via pendant drop or tensiometry .
Advanced methods like LC-MS provide structural elucidation, while AFM/SEM assess surface morphology in corrosion studies .
Q. Advanced: How can researchers resolve contradictions in optimal molar ratios for diethanolamide synthesis?
Answer:
Discrepancies arise from differing goals:
- 1:1 ratios (oleic acid:DEA) minimize free DEA, favoring high-purity amides for cosmetics .
- 1:2 ratios may be used industrially for cost efficiency but require post-synthesis purification .
Methodological Approach : - Conduct kinetic studies to track byproduct formation (e.g., esters) .
- Use DOE (Design of Experiments) to optimize ratios for specific applications (e.g., surfactants vs. lubricants) .
Q. Advanced: What experimental design considerations are critical when evaluating this compound’s efficacy in novel applications (e.g., enhanced oil recovery or corrosion inhibition)?
Answer:
Key factors include:
- Surfactant performance : Measure interfacial tension (IFT) at water/oil interfaces; this compound achieves IFT <1.0 mN/m, critical for oil displacement .
- Corrosion inhibition : Use electrochemical impedance spectroscopy (EIS) and mass loss assays in acidic media. CDEA (coconut diethanolamide) shows >80% inhibition efficiency on steel in trichloroacetic acid .
- Lubrication : Compare wear scar radii via four-ball tests; this compound’s hydroxyl groups reduce friction by 78.2% .
Data Contradiction Example : - Hydroxyl-rich surfactants may underperform vs. amine-rich analogs in extreme pressure (e.g., oleic diethylenetriamine reduces friction by 83.6%) .
Q. Basic: How does this compound’s molecular structure contribute to its surfactant and antistatic properties?
Answer:
The molecule’s polar head (diethanolamide) and nonpolar tail (oleic acid) enable:
- Micelle formation : Lowers surface tension in aqueous solutions (e.g., 25–35 mN/m) .
- Antistatic effects : Hydrophilic groups dissipate static charge in polymers and textiles .
- Emulsification : Stabilizes oil-water interfaces in metalworking fluids .
Q. Advanced: What challenges arise in scaling up enzymatic synthesis of this compound, and how can they be mitigated?
Answer:
Challenges :
- Enzyme stability: Lipases denature at high temperatures (>70°C) .
- Viscosity: Excess DEA forms ion pairs, hindering mixing .
Solutions : - Immobilize enzymes on supports (e.g., Na alginate) for reusability .
- Gradual DEA addition and solvent-free systems reduce viscosity .
Q. Basic: What are the regulatory and safety considerations for handling this compound in lab settings?
Answer:
- Toxicity : Classified as non-TSCA for industrial use; avoid inhalation and skin contact .
- Regulatory compliance : Follow EU Cosmetic Ingredient (INCI) guidelines for purity in personal care products .
Q. Advanced: How can computational modeling (e.g., DFT) elucidate this compound’s adsorption mechanisms on metal surfaces?
Answer:
相似化合物的比较
Comparison with Similar Diethanolamide Surfactants
Below is a detailed comparison of ODEA with structurally analogous compounds, focusing on alkyl chain saturation, functional properties, and applications.
Table 1: Structural and Functional Comparison
Key Findings:
Alkyl Chain Saturation and Stability: Saturated derivatives (e.g., stearic diethanolamide) exhibit higher thermal stability and are suited for solid formulations . Unsaturated variants (e.g., ODEA, linthis compound) offer better solubility and interfacial activity due to kinked alkyl chains . Hydroxylated ricinthis compound demonstrates unique hydrophilic-lipophilic balance (HLB) for specialized emulsification .
Functional Performance: ODEA outperforms coconut-based diethanolamides in calcium/magnesium dispersion, making it effective in hard water . Linthis compound’s isomerism may influence biological interactions, as seen in preeclampsia biomarker studies .
Synthesis Efficiency: ODEA achieves 61–65% conversion in solvent-free systems, rising to 78% with n-hexane .
Industrial and Environmental Considerations
- Biodegradability : ODEA is biodegradable, aligning with eco-friendly surfactant trends .
- Safety: NTP dermal studies on rats/mice classified ODEA as non-carcinogenic but noted mild skin irritation .
- Market Trends : ODEA is pivotal in the expanding personal care and industrial lubricant sectors, with key manufacturers including Stepan and Solvay .
属性
IUPAC Name |
(Z)-N,N-bis(2-hydroxyethyl)octadec-9-enamide | |
---|---|---|
Source | PubChem | |
URL | https://pubchem.ncbi.nlm.nih.gov | |
Description | Data deposited in or computed by PubChem | |
InChI |
InChI=1S/C22H43NO3/c1-2-3-4-5-6-7-8-9-10-11-12-13-14-15-16-17-22(26)23(18-20-24)19-21-25/h9-10,24-25H,2-8,11-21H2,1H3/b10-9- | |
Source | PubChem | |
URL | https://pubchem.ncbi.nlm.nih.gov | |
Description | Data deposited in or computed by PubChem | |
InChI Key |
LPMBTLLQQJBUOO-KTKRTIGZSA-N | |
Source | PubChem | |
URL | https://pubchem.ncbi.nlm.nih.gov | |
Description | Data deposited in or computed by PubChem | |
Canonical SMILES |
CCCCCCCCC=CCCCCCCCC(=O)N(CCO)CCO | |
Source | PubChem | |
URL | https://pubchem.ncbi.nlm.nih.gov | |
Description | Data deposited in or computed by PubChem | |
Isomeric SMILES |
CCCCCCCC/C=C\CCCCCCCC(=O)N(CCO)CCO | |
Source | PubChem | |
URL | https://pubchem.ncbi.nlm.nih.gov | |
Description | Data deposited in or computed by PubChem | |
Molecular Formula |
C22H43NO3 | |
Source | PubChem | |
URL | https://pubchem.ncbi.nlm.nih.gov | |
Description | Data deposited in or computed by PubChem | |
DSSTOX Substance ID |
DTXSID8026563 | |
Record name | Oleic acid diethanolamide | |
Source | EPA DSSTox | |
URL | https://comptox.epa.gov/dashboard/DTXSID8026563 | |
Description | DSSTox provides a high quality public chemistry resource for supporting improved predictive toxicology. | |
Molecular Weight |
369.6 g/mol | |
Source | PubChem | |
URL | https://pubchem.ncbi.nlm.nih.gov | |
Description | Data deposited in or computed by PubChem | |
Physical Description |
Liquid | |
Record name | 9-Octadecenamide, N,N-bis(2-hydroxyethyl)-, (9Z)- | |
Source | EPA Chemicals under the TSCA | |
URL | https://www.epa.gov/chemicals-under-tsca | |
Description | EPA Chemicals under the Toxic Substances Control Act (TSCA) collection contains information on chemicals and their regulations under TSCA, including non-confidential content from the TSCA Chemical Substance Inventory and Chemical Data Reporting. | |
CAS No. |
93-83-4 | |
Record name | Oleic acid diethanolamide | |
Source | CAS Common Chemistry | |
URL | https://commonchemistry.cas.org/detail?cas_rn=93-83-4 | |
Description | CAS Common Chemistry is an open community resource for accessing chemical information. Nearly 500,000 chemical substances from CAS REGISTRY cover areas of community interest, including common and frequently regulated chemicals, and those relevant to high school and undergraduate chemistry classes. This chemical information, curated by our expert scientists, is provided in alignment with our mission as a division of the American Chemical Society. | |
Explanation | The data from CAS Common Chemistry is provided under a CC-BY-NC 4.0 license, unless otherwise stated. | |
Record name | Oleic acid diethanolamide | |
Source | ChemIDplus | |
URL | https://pubchem.ncbi.nlm.nih.gov/substance/?source=chemidplus&sourceid=0000093834 | |
Description | ChemIDplus is a free, web search system that provides access to the structure and nomenclature authority files used for the identification of chemical substances cited in National Library of Medicine (NLM) databases, including the TOXNET system. | |
Record name | 9-Octadecenamide, N,N-bis(2-hydroxyethyl)-, (9Z)- | |
Source | EPA Chemicals under the TSCA | |
URL | https://www.epa.gov/chemicals-under-tsca | |
Description | EPA Chemicals under the Toxic Substances Control Act (TSCA) collection contains information on chemicals and their regulations under TSCA, including non-confidential content from the TSCA Chemical Substance Inventory and Chemical Data Reporting. | |
Record name | Oleic acid diethanolamide | |
Source | EPA DSSTox | |
URL | https://comptox.epa.gov/dashboard/DTXSID8026563 | |
Description | DSSTox provides a high quality public chemistry resource for supporting improved predictive toxicology. | |
Record name | Amides, C18-unsatd., N,N-bis(hydroxyethyl) | |
Source | European Chemicals Agency (ECHA) | |
URL | https://echa.europa.eu/substance-information/-/substanceinfo/100.233.053 | |
Description | The European Chemicals Agency (ECHA) is an agency of the European Union which is the driving force among regulatory authorities in implementing the EU's groundbreaking chemicals legislation for the benefit of human health and the environment as well as for innovation and competitiveness. | |
Explanation | Use of the information, documents and data from the ECHA website is subject to the terms and conditions of this Legal Notice, and subject to other binding limitations provided for under applicable law, the information, documents and data made available on the ECHA website may be reproduced, distributed and/or used, totally or in part, for non-commercial purposes provided that ECHA is acknowledged as the source: "Source: European Chemicals Agency, http://echa.europa.eu/". Such acknowledgement must be included in each copy of the material. ECHA permits and encourages organisations and individuals to create links to the ECHA website under the following cumulative conditions: Links can only be made to webpages that provide a link to the Legal Notice page. | |
Record name | N,N-bis(2-hydroxyethyl)oleamide | |
Source | European Chemicals Agency (ECHA) | |
URL | https://echa.europa.eu/substance-information/-/substanceinfo/100.002.075 | |
Description | The European Chemicals Agency (ECHA) is an agency of the European Union which is the driving force among regulatory authorities in implementing the EU's groundbreaking chemicals legislation for the benefit of human health and the environment as well as for innovation and competitiveness. | |
Explanation | Use of the information, documents and data from the ECHA website is subject to the terms and conditions of this Legal Notice, and subject to other binding limitations provided for under applicable law, the information, documents and data made available on the ECHA website may be reproduced, distributed and/or used, totally or in part, for non-commercial purposes provided that ECHA is acknowledged as the source: "Source: European Chemicals Agency, http://echa.europa.eu/". Such acknowledgement must be included in each copy of the material. ECHA permits and encourages organisations and individuals to create links to the ECHA website under the following cumulative conditions: Links can only be made to webpages that provide a link to the Legal Notice page. | |
Record name | OLEIC DIETHANOLAMIDE | |
Source | FDA Global Substance Registration System (GSRS) | |
URL | https://gsrs.ncats.nih.gov/ginas/app/beta/substances/5C1I3E441Q | |
Description | The FDA Global Substance Registration System (GSRS) enables the efficient and accurate exchange of information on what substances are in regulated products. Instead of relying on names, which vary across regulatory domains, countries, and regions, the GSRS knowledge base makes it possible for substances to be defined by standardized, scientific descriptions. | |
Explanation | Unless otherwise noted, the contents of the FDA website (www.fda.gov), both text and graphics, are not copyrighted. They are in the public domain and may be republished, reprinted and otherwise used freely by anyone without the need to obtain permission from FDA. Credit to the U.S. Food and Drug Administration as the source is appreciated but not required. | |
Retrosynthesis Analysis
AI-Powered Synthesis Planning: Our tool employs the Template_relevance Pistachio, Template_relevance Bkms_metabolic, Template_relevance Pistachio_ringbreaker, Template_relevance Reaxys, Template_relevance Reaxys_biocatalysis model, leveraging a vast database of chemical reactions to predict feasible synthetic routes.
One-Step Synthesis Focus: Specifically designed for one-step synthesis, it provides concise and direct routes for your target compounds, streamlining the synthesis process.
Accurate Predictions: Utilizing the extensive PISTACHIO, BKMS_METABOLIC, PISTACHIO_RINGBREAKER, REAXYS, REAXYS_BIOCATALYSIS database, our tool offers high-accuracy predictions, reflecting the latest in chemical research and data.
Strategy Settings
Precursor scoring | Relevance Heuristic |
---|---|
Min. plausibility | 0.01 |
Model | Template_relevance |
Template Set | Pistachio/Bkms_metabolic/Pistachio_ringbreaker/Reaxys/Reaxys_biocatalysis |
Top-N result to add to graph | 6 |
Feasible Synthetic Routes
体外研究产品的免责声明和信息
请注意,BenchChem 上展示的所有文章和产品信息仅供信息参考。 BenchChem 上可购买的产品专为体外研究设计,这些研究在生物体外进行。体外研究,源自拉丁语 "in glass",涉及在受控实验室环境中使用细胞或组织进行的实验。重要的是要注意,这些产品没有被归类为药物或药品,他们没有得到 FDA 的批准,用于预防、治疗或治愈任何医疗状况、疾病或疾病。我们必须强调,将这些产品以任何形式引入人类或动物的身体都是法律严格禁止的。遵守这些指南对确保研究和实验的法律和道德标准的符合性至关重要。