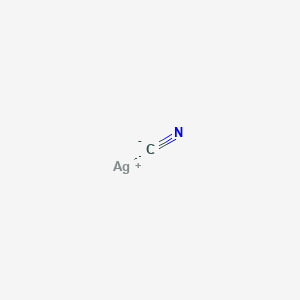
氰化银
描述
Silver cyanide is a chemical compound with the formula AgCN. It is a white, odorless, and tasteless powder that darkens upon exposure to light. This compound is insoluble in water but soluble in aqueous ammonia, dilute boiling nitric acid, aqueous potassium cyanide solution, and aqueous sodium thiosulfate solutions . Silver cyanide is primarily used in silver-plating processes .
作用机制
Target of Action
Silver cyanide primarily targets the cytochrome C oxidase in the mitochondria of cells . This enzyme plays a crucial role in the electron transport chain of aerobic cellular respiration .
Mode of Action
The cyanide ion in silver cyanide binds to the iron atom in cytochrome C oxidase, acting as an irreversible enzyme inhibitor . This prevents cytochrome C oxidase from transporting electrons to oxygen, disrupting the electron transport chain . The silver ion in silver cyanide forms linear two-coordinate Ag+ ions bridged by the cyanide ions .
Biochemical Pathways
The binding of cyanide to cytochrome C oxidase disrupts the electron transport chain, a crucial part of aerobic cellular respiration . This disruption prevents cells from using oxygen, leading to rapid cell death .
Pharmacokinetics
It’s known that silver ions are highly reactive and can form complexes with proteins and other biological molecules . The toxicity and bioavailability of silver cyanide are likely influenced by these interactions.
Result of Action
The primary result of silver cyanide’s action is cell death due to the disruption of aerobic cellular respiration . This occurs because the cyanide ion prevents cells from using oxygen by inhibiting cytochrome C oxidase .
Action Environment
Environmental factors can influence the action of silver cyanide. For instance, the presence of other metal ions can affect the reactivity and toxicity of silver cyanide . Additionally, the pH and temperature of the environment can influence the solubility and reactivity of silver cyanide .
科学研究应用
Silver cyanide has several applications in scientific research and industry:
Silver-Plating: It is widely used in the electroplating industry to deposit a thin layer of silver on various substrates.
Analytical Chemistry: Silver cyanide is used in analytical chemistry for the detection and quantification of cyanide ions.
Material Science: It is used in the preparation of luminescent materials such as thallium silver cyanide complexes.
Nanotechnology: Silver cyanide nanoparticles are used in the development of sensors for detecting cyanide in water.
生化分析
Biochemical Properties
Silver cyanide is known to interact with various biomolecules. For instance, it can bind to the iron atom in cytochrome C oxidase in the mitochondria of cells . This binding can inhibit the enzyme, preventing it from transporting electrons to oxygen in the electron transport chain of aerobic cellular respiration .
Cellular Effects
The binding of silver cyanide to cytochrome C oxidase can have significant effects on cell function. It can prevent cells from using oxygen to make energy molecules . This can impact cell signaling pathways, gene expression, and cellular metabolism .
Molecular Mechanism
The molecular mechanism of silver cyanide involves its binding to biomolecules such as the iron atom in cytochrome C oxidase . This binding can inhibit the enzyme, preventing it from doing its job, which is to transport electrons to oxygen in the electron transport chain of aerobic cellular respiration .
Temporal Effects in Laboratory Settings
The effects of silver cyanide can change over time in laboratory settings. For instance, silver cyanide is known for its role in the undesirable browning of plant products . The reaction product of silver cyanide, o-quinones, are responsible for the formation of dark brown coloration of plant products .
Dosage Effects in Animal Models
The effects of silver cyanide can vary with different dosages in animal models. For instance, the lethal dosage of hydrogen cyanide (HCN) in most animal species is 2 mg/kg . Plant materials containing ≥200 ppm of cyanogenic glycosides are dangerous .
Metabolic Pathways
Silver cyanide can be involved in various metabolic pathways. For instance, cyanide, a component of silver cyanide, can disrupt iodide uptake by the follicular thyroid cell sodium-iodide symporter by thiocyanate, a metabolite in the detoxification of cyanide .
Transport and Distribution
Silver cyanide can be transported and distributed within cells and tissues. All elements of the cyanide transportation and distribution system bringing cyanide from its point of manufacture to a gold or silver mining operation are subject to this Protocol .
Subcellular Localization
It is known that cyanide, a component of silver cyanide, can bind to the iron atom in cytochrome C oxidase in the mitochondria of cells .
准备方法
Synthetic Routes and Reaction Conditions: Silver cyanide can be synthesized by reacting silver nitrate with sodium cyanide. The reaction is as follows:
AgNO3+NaCN→AgCN+NaNO3
This reaction results in the precipitation of silver cyanide as a white solid .
Industrial Production Methods: In industrial settings, silver cyanide is often produced by the reaction of silver nitrate with potassium cyanide. The process involves dissolving silver nitrate in water and then adding potassium cyanide solution under controlled conditions to precipitate silver cyanide. The precipitate is then filtered, washed, and dried .
Types of Reactions:
Oxidation and Reduction: Silver cyanide can undergo oxidation and reduction reactions. For example, it can be reduced to metallic silver by heating in the presence of a reducing agent.
Silver cyanide reacts with halides to form silver halides and cyanide ions. For instance:Substitution: AgCN+Cl−→AgCl+CN−
Silver cyanide forms complexes with various ligands such as ammonia and tertiary phosphines. For example:Complex Formation: AgCN+2NH3→[Ag(NH3)2]++CN−
Common Reagents and Conditions:
Oxidizing Agents: Hydrogen peroxide and vanadium pentoxide can be used as oxidizing agents in reactions involving silver cyanide.
Reducing Agents: Zinc and other reducing metals can reduce silver cyanide to metallic silver.
Major Products Formed:
Metallic Silver: Reduction of silver cyanide results in the formation of metallic silver.
Silver Halides: Reaction with halides forms silver halides such as silver chloride.
相似化合物的比较
Sodium Cyanide (NaCN): Sodium cyanide is a highly soluble cyanide salt used in gold mining and electroplating.
Potassium Cyanide (KCN): Potassium cyanide is similar to sodium cyanide in its applications and toxicity.
Copper(I) Cyanide (CuCN): Copper(I) cyanide is used in electroplating and as a catalyst in organic synthesis.
Uniqueness of Silver Cyanide: Silver cyanide is unique due to its specific use in silver-plating and its ability to form stable complexes with various ligands. Its insolubility in water and stability under certain conditions make it suitable for specific industrial applications .
属性
IUPAC Name |
silver;cyanide | |
---|---|---|
Details | Computed by LexiChem 2.6.6 (PubChem release 2019.06.18) | |
Source | PubChem | |
URL | https://pubchem.ncbi.nlm.nih.gov | |
Description | Data deposited in or computed by PubChem | |
InChI |
InChI=1S/CN.Ag/c1-2;/q-1;+1 | |
Details | Computed by InChI 1.0.5 (PubChem release 2019.06.18) | |
Source | PubChem | |
URL | https://pubchem.ncbi.nlm.nih.gov | |
Description | Data deposited in or computed by PubChem | |
InChI Key |
LFAGQMCIGQNPJG-UHFFFAOYSA-N | |
Details | Computed by InChI 1.0.5 (PubChem release 2019.06.18) | |
Source | PubChem | |
URL | https://pubchem.ncbi.nlm.nih.gov | |
Description | Data deposited in or computed by PubChem | |
Canonical SMILES |
[C-]#N.[Ag+] | |
Details | Computed by OEChem 2.1.5 (PubChem release 2019.06.18) | |
Source | PubChem | |
URL | https://pubchem.ncbi.nlm.nih.gov | |
Description | Data deposited in or computed by PubChem | |
Molecular Formula |
CAgN | |
Details | Computed by PubChem 2.1 (PubChem release 2019.06.18) | |
Source | PubChem | |
URL | https://pubchem.ncbi.nlm.nih.gov | |
Description | Data deposited in or computed by PubChem | |
DSSTOX Substance ID |
DTXSID9024306 | |
Record name | Silver cyanide | |
Source | EPA DSSTox | |
URL | https://comptox.epa.gov/dashboard/DTXSID9024306 | |
Description | DSSTox provides a high quality public chemistry resource for supporting improved predictive toxicology. | |
Molecular Weight |
133.886 g/mol | |
Details | Computed by PubChem 2.1 (PubChem release 2021.05.07) | |
Source | PubChem | |
URL | https://pubchem.ncbi.nlm.nih.gov | |
Description | Data deposited in or computed by PubChem | |
CAS No. |
506-64-9 | |
Record name | Silver cyanide | |
Source | EPA DSSTox | |
URL | https://comptox.epa.gov/dashboard/DTXSID9024306 | |
Description | DSSTox provides a high quality public chemistry resource for supporting improved predictive toxicology. | |
Record name | SILVER CYANIDE | |
Source | FDA Global Substance Registration System (GSRS) | |
URL | https://gsrs.ncats.nih.gov/ginas/app/beta/substances/33RV6XQ01M | |
Description | The FDA Global Substance Registration System (GSRS) enables the efficient and accurate exchange of information on what substances are in regulated products. Instead of relying on names, which vary across regulatory domains, countries, and regions, the GSRS knowledge base makes it possible for substances to be defined by standardized, scientific descriptions. | |
Explanation | Unless otherwise noted, the contents of the FDA website (www.fda.gov), both text and graphics, are not copyrighted. They are in the public domain and may be republished, reprinted and otherwise used freely by anyone without the need to obtain permission from FDA. Credit to the U.S. Food and Drug Administration as the source is appreciated but not required. | |
Retrosynthesis Analysis
AI-Powered Synthesis Planning: Our tool employs the Template_relevance Pistachio, Template_relevance Bkms_metabolic, Template_relevance Pistachio_ringbreaker, Template_relevance Reaxys, Template_relevance Reaxys_biocatalysis model, leveraging a vast database of chemical reactions to predict feasible synthetic routes.
One-Step Synthesis Focus: Specifically designed for one-step synthesis, it provides concise and direct routes for your target compounds, streamlining the synthesis process.
Accurate Predictions: Utilizing the extensive PISTACHIO, BKMS_METABOLIC, PISTACHIO_RINGBREAKER, REAXYS, REAXYS_BIOCATALYSIS database, our tool offers high-accuracy predictions, reflecting the latest in chemical research and data.
Strategy Settings
Precursor scoring | Relevance Heuristic |
---|---|
Min. plausibility | 0.01 |
Model | Template_relevance |
Template Set | Pistachio/Bkms_metabolic/Pistachio_ringbreaker/Reaxys/Reaxys_biocatalysis |
Top-N result to add to graph | 6 |
Feasible Synthetic Routes
Q1: What is the molecular formula and weight of silver cyanide?
A1: Silver cyanide has the molecular formula AgCN, with a molecular weight of 133.886 g/mol.
Q2: What does solid-state NMR reveal about the structure of silver cyanide?
A2: Solid-state (13)C and (15)N NMR spectroscopy studies [] reveal that silver cyanide exists as linear polymeric chains (-Ag-CN-)n. Interestingly, these studies identified a degree of disorder within the chains, with approximately 30% of silver sites showing reversed cyanide orientation (-NC-Ag-CN- or -CN-Ag-NC-) and 70% exhibiting an ordered arrangement (-NC-Ag-NC-).
Q3: Can you elaborate on the bonding and structure of silver cyanide complexes with thiourea and its derivatives?
A3: Research has explored the crystal structures of silver cyanide complexes with various sulfur-containing ligands []. These complexes, which include thiourea (tu), 1-methyl-2-thiourea (mtu), and others, demonstrate polymeric structures (one- or two-dimensional) stabilized by bridging sulfur and cyanide groups. Interestingly, the specific arrangement varies depending on the ligand, highlighting the impact of ligand structure on complex formation.
Q4: What is the role of potassium ions in modifying the luminescence of silver cyanide complexes?
A4: Introducing potassium ions into silver cyanide complexes can yield heterometallic structures with intriguing luminescent properties []. For instance, [Me4N]2[KAg3(CN)6] displays a NaCl-type framework with ligand-unsupported argentophilic interactions and exhibits green luminescence. Another complex, [Ag3(H2O)3][K(CN)2]3, forms a unique 3D framework with [Ag3(H2O)3] clusters connecting [K(CN)2] layers, also resulting in green luminescence. These findings demonstrate the potential for tuning the luminescent behavior of silver cyanide complexes through the incorporation of potassium ions.
Q5: How does the presence of oxygen affect the adsorption of silver cyanide onto activated carbon?
A5: Studies on the adsorption of silver cyanide onto activated carbon [, ] show that oxygen plays a crucial role. Increasing dissolved oxygen levels initially enhance the adsorption of silver cyanide, but this effect plateaus beyond a certain oxygen concentration. This suggests a two-fold adsorption mechanism, with one pathway involving oxygen consumption for active site oxidation and the other proceeding without oxygen involvement.
Q6: What challenges can arise from the formation of silver cyanide on silver objects, and how can these be addressed?
A6: The presence of silver cyanide on silver artifacts, potentially formed during cleaning or re-plating processes [, ], poses a challenge due to its toxicity and light sensitivity. Treatment with a 20% sodium thiosulfate solution has proven effective in removing silver cyanide, ensuring the safe handling and preservation of such objects.
Q7: How is silver cyanide utilized in the preparation of hindered esters?
A7: Silver cyanide acts as a catalyst in the synthesis of sterically hindered esters from acyl chlorides and alcohols []. This method offers advantages over traditional approaches, such as the use of pyridine, in terms of reaction rate and yield, particularly for sterically demanding substrates.
Q8: Can you describe the role of silver cyanide in the selective epoxidation of cyclohexene and styrene?
A8: Research demonstrates the effectiveness of silver cyanide powder as a catalyst for the selective epoxidation of cyclohexene and styrene []. This process, conducted in acetonitrile with hydrogen peroxide as the oxidant, showcases high selectivity towards the corresponding epoxides. Notably, the silver cyanide catalyst can be recovered and reused, indicating its potential for sustainable catalytic applications.
Q9: How does silver cyanide participate in the Reissert reaction of 4,6-phenanthroline?
A9: Silver cyanide plays a key role in the Reissert reaction of 4,6-phenanthroline [], facilitating the formation of various Reissert compounds. This reaction, which involves the addition of a cyanide group and an acyl group across a carbon-nitrogen double bond, highlights the versatility of silver cyanide in organic synthesis.
Q10: How can silver cyanide be prepared for industrial applications?
A10: High-purity silver cyanide for industrial applications, like silver plating, can be produced through several methods. One approach involves reacting silver nitrate with potassium cyanide [, , ]. Controlling the reaction conditions, such as the molar ratio of reactants, stirring rate, and temperature, are critical for obtaining high-purity silver cyanide with desirable color and crystal properties.
Q11: How can ICP-AES be utilized to analyze silver cyanide solutions?
A11: Inductively Coupled Plasma Atomic Emission Spectrometry (ICP-AES) proves valuable in analyzing the composition of silver cyanide solutions [, ]. This technique enables the accurate determination of impurity elements, such as copper, iron, lead, nickel, zinc, and antimony, in silver cyanide baths used for electroplating. By monitoring these impurities, manufacturers can maintain the quality and effectiveness of their plating processes.
Q12: How can a capillary ion analyzer be employed to monitor the composition of silver plating baths?
A12: A capillary ion analyzer offers a powerful tool for the simultaneous analysis of various components in silver plating baths [, ]. This technique, utilizing a chromate buffer at pH 11 and on-column indirect photometry at 254 nm, allows for the quantification of potassium silver cyanide, phosphate, free cyanide, and borate salts. This capability is crucial for optimizing bath performance and ensuring consistent plating results.
Q13: What safety precautions should be taken when handling silver cyanide?
A13: Silver cyanide is a highly toxic compound. It is crucial to handle it with extreme care, using appropriate personal protective equipment such as gloves and respirators. Direct contact and inhalation should be strictly avoided.
Q14: How can silver be recovered from dilute cyanide solutions in an environmentally friendly way?
A14: Electrogenerative processes offer a promising route for the environmentally sound recovery of silver from dilute cyanide solutions []. This approach involves using a closed-loop system where silver is deposited on a reticulated vitreous carbon electrode, allowing for the recovery and reuse of the cyanide solution. This method presents a sustainable alternative to traditional methods, minimizing waste and environmental impact.
Q15: What are some insights into the degradation of silver cyanide by the hydroxyl radical?
A15: Computational and experimental studies have explored the oxidation of silver cyanide by the hydroxyl radical in aqueous solutions []. Ab initio calculations and pulse radiolysis experiments provided evidence for the formation of an oxidized silver cyanide complex, AgII(CN)2(H2O)2. This research sheds light on the fate of silver cyanide in environmental systems and its potential degradation pathways.
Q16: Are there alternative adsorbents to activated carbon for recovering silver from cyanide solutions?
A16: Yes, research has focused on developing alternative adsorbents for recovering silver from cyanide solutions. One such alternative is a chelate resin containing sulfur and nitrogen []. This resin selectively adsorbs silver cyanide, which can then be recovered using a 2% NaCN solution. This method avoids the use of activated carbon and offers a potentially more sustainable approach to silver recovery.
Q17: What historical research has been done on the electrochemical behavior of silver anodes in cyanide baths?
A17: Early research on silver electroplating explored the behavior of silver anodes in cyanide baths []. These studies identified a phenomenon termed "mechanical passivation," where a layer of silver oxide and silver cyanide forms on the anode surface, hindering further dissolution. Understanding the factors influencing this passivation, such as free cyanide concentration, is critical for optimizing silver plating processes.
体外研究产品的免责声明和信息
请注意,BenchChem 上展示的所有文章和产品信息仅供信息参考。 BenchChem 上可购买的产品专为体外研究设计,这些研究在生物体外进行。体外研究,源自拉丁语 "in glass",涉及在受控实验室环境中使用细胞或组织进行的实验。重要的是要注意,这些产品没有被归类为药物或药品,他们没有得到 FDA 的批准,用于预防、治疗或治愈任何医疗状况、疾病或疾病。我们必须强调,将这些产品以任何形式引入人类或动物的身体都是法律严格禁止的。遵守这些指南对确保研究和实验的法律和道德标准的符合性至关重要。