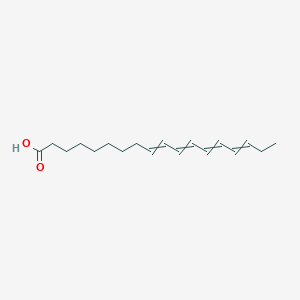
β-巴豆酸
- 点击 快速询问 获取最新报价。
- 提供有竞争力价格的高质量产品,您可以更专注于研究。
描述
Parinaric acid is an octadecatetraenoic acid containing a conjugated system of double bonds at positions 9, 11, 13 and 15.
Parinaric acid is a natural product found in Impatiens balsamina with data available.
Parinaric Acid is a polyunsaturated long-chain fatty acid with an 18-carbon backbone and 4 double bonds originating from the 3rd, 5th, 7th and 9th positions from the methyl end.
科学研究应用
作用机制
Target of Action
Beta-Parinaric acid, a naturally occurring fluorescent compound, is primarily used as a molecular probe of lipid-lipid interactions . It integrates normally into the phospholipid bilayer of mammalian cells , serving as a key player in monitoring phase transitions in bilayer lipid membranes .
Mode of Action
The mode of action of beta-Parinaric acid involves its interaction with lipid membranes. It is incorporated into the membranes or isolated membrane lipids, revealing characteristic breaks or changes in slope with both the plasma membranes and their extracted lipids . These parameters are measured as a function of temperature .
Biochemical Pathways
Beta-Parinaric acid is involved in cross-linked biochemical pathways, including purine, histidine, and polyamine metabolism . It is synthesized from α-linolenic acid . The enzyme responsible for the creation of the conjugated double bonds in Parinaric acid is identified as a “conjugase”, which is related to the family of fatty acid desaturase enzymes responsible for putting double bonds into fatty acids .
Pharmacokinetics
The pharmacokinetics of beta-Parinaric acid, like other drugs, involves the processes of absorption, distribution, metabolism, and elimination . .
Result of Action
The result of beta-Parinaric acid’s action is primarily observed in the changes it induces in the lipid membranes. It reveals characteristic breaks or changes in slope with both the plasma membranes and their extracted lipids . These changes are indicative of the compound’s influence on the structural and functional dynamics of the membranes.
Action Environment
The action of beta-Parinaric acid is influenced by environmental factors such as temperature . Its effects on plasma membranes, microsomes, and mitochondria, as well as on their respective lipids, are measured as a function of temperature . This suggests that the compound’s action, efficacy, and stability may vary under different environmental conditions.
属性
{ "Design of the Synthesis Pathway": "The synthesis of beta-Parinaric acid can be achieved through a multi-step process involving several chemical reactions.", "Starting Materials": [ "2-methyl-2-butene", "ozone", "methanol", "sodium borohydride", "acetic acid", "hydrochloric acid", "sodium hydroxide", "sulfuric acid", "potassium permanganate", "sodium sulfite", "sodium bicarbonate", "sodium chloride", "beta-carotene" ], "Reaction": [ "Step 1: Ozonolysis of 2-methyl-2-butene to form 2-methyl-2-butene-1,4-dial", "Step 2: Reduction of 2-methyl-2-butene-1,4-dial with sodium borohydride in methanol to form 2-methyl-2-butene-1,4-diol", "Step 3: Oxidation of 2-methyl-2-butene-1,4-diol with potassium permanganate in sulfuric acid to form 2-methyl-2-butene-1,4-dicarboxylic acid", "Step 4: Conversion of 2-methyl-2-butene-1,4-dicarboxylic acid to beta-Parinaric acid via the following reactions:", "- Reaction 1: Esterification of 2-methyl-2-butene-1,4-dicarboxylic acid with methanol in the presence of sulfuric acid to form methyl 2-methyl-2-butene-1,4-dicarboxylate", "- Reaction 2: Hydrolysis of methyl 2-methyl-2-butene-1,4-dicarboxylate with sodium hydroxide to form 2-methyl-2-butene-1,4-dicarboxylic acid", "- Reaction 3: Decarboxylation of 2-methyl-2-butene-1,4-dicarboxylic acid with sodium bicarbonate to form beta-Parinaric acid", "Step 5: Purification of beta-Parinaric acid by recrystallization from a solvent such as ethanol or methanol" ] } | |
CAS 编号 |
18841-21-9 |
分子式 |
C18H28O2 |
分子量 |
276.4 g/mol |
IUPAC 名称 |
octadeca-9,11,13,15-tetraenoic acid |
InChI |
InChI=1S/C18H28O2/c1-2-3-4-5-6-7-8-9-10-11-12-13-14-15-16-17-18(19)20/h3-10H,2,11-17H2,1H3,(H,19,20) |
InChI 键 |
IJTNSXPMYKJZPR-UHFFFAOYSA-N |
手性 SMILES |
CC/C=C/C=C/C=C/C=C/CCCCCCCC(=O)O |
SMILES |
CCC=CC=CC=CC=CCCCCCCCC(=O)O |
规范 SMILES |
CCC=CC=CC=CC=CCCCCCCCC(=O)O |
18841-21-9 | |
Pictograms |
Irritant |
同义词 |
(all-E)-9,11,13,15-Octadecatetraenoic Acid; 9-trans,11-trans,13-trans,15-trans-Octadecatetraenoic Acid; trans-Parinaric Acid; β-PAC; β-Parinaric Acid |
产品来源 |
United States |
Retrosynthesis Analysis
AI-Powered Synthesis Planning: Our tool employs the Template_relevance Pistachio, Template_relevance Bkms_metabolic, Template_relevance Pistachio_ringbreaker, Template_relevance Reaxys, Template_relevance Reaxys_biocatalysis model, leveraging a vast database of chemical reactions to predict feasible synthetic routes.
One-Step Synthesis Focus: Specifically designed for one-step synthesis, it provides concise and direct routes for your target compounds, streamlining the synthesis process.
Accurate Predictions: Utilizing the extensive PISTACHIO, BKMS_METABOLIC, PISTACHIO_RINGBREAKER, REAXYS, REAXYS_BIOCATALYSIS database, our tool offers high-accuracy predictions, reflecting the latest in chemical research and data.
Strategy Settings
Precursor scoring | Relevance Heuristic |
---|---|
Min. plausibility | 0.01 |
Model | Template_relevance |
Template Set | Pistachio/Bkms_metabolic/Pistachio_ringbreaker/Reaxys/Reaxys_biocatalysis |
Top-N result to add to graph | 6 |
Feasible Synthetic Routes
Q1: How does beta-Parinaric acid interact with membranes and what are the downstream effects of this interaction?
A1: beta-Parinaric acid is a naturally occurring conjugated polyene fatty acid that exhibits fluorescence, making it a useful probe for studying membrane structure and dynamics. [, ] Its hydrophobic nature allows it to readily incorporate into the lipophilic environment of lipid bilayers. [, ] This incorporation doesn't significantly disrupt membrane structure, allowing for observations of the membrane in a near-native state. The fluorescence properties of beta-Parinaric acid are sensitive to the surrounding environment, particularly the fluidity and order of the lipid molecules. [, ] Changes in temperature, lipid composition, or the presence of membrane-interacting molecules can alter the fluorescence intensity and polarization of beta-Parinaric acid, providing insights into membrane phase transitions, fluidity changes, and lipid-protein interactions. [, , ]
Q2: Can you provide the structural characterization of beta-Parinaric acid, including its molecular formula, weight, and spectroscopic data?
A2: * Molecular Formula: C18H28O2* Molecular Weight: 288.4 g/mol* Spectroscopic Data: * UV-Vis Absorption: Maximum absorbance around 300-320 nm due to the conjugated tetraene system. [, ] * Fluorescence Emission: Maximum emission around 420-440 nm when excited near its absorption maximum. [, ]
Q3: How is beta-Parinaric acid used to study membrane phase transitions?
A3: beta-Parinaric acid exhibits a change in its fluorescence properties in response to alterations in membrane fluidity, which is directly related to phase transitions. [, ] When incorporated into artificial lipid bilayers, such as those composed of dimyristoyl phosphatidylcholine, dipalmitoyl phosphatidylcholine, or distearoyl phosphatidylcholine, a sharp change in beta-Parinaric acid fluorescence intensity is observed at the characteristic phase transition temperature of each lipid. [] This allows researchers to pinpoint the temperature at which the membrane transitions between a more ordered gel phase and a more fluid liquid crystalline phase. [, ]
Q4: How does the presence of cholesterol affect the membrane properties studied using beta-Parinaric acid?
A4: Studies utilizing beta-Parinaric acid demonstrate that the addition of cholesterol to lipid bilayers significantly affects the membrane phase transition. [] Cholesterol, with its rigid sterol ring structure, disrupts the regular packing of phospholipid acyl chains, leading to a broadening of the phase transition and a decrease in its magnitude as measured by fluorescence intensity changes of beta-Parinaric acid. [] This suggests that cholesterol increases membrane fluidity at lower temperatures while decreasing fluidity at higher temperatures, effectively creating a more stable membrane environment less susceptible to dramatic phase shifts. []
Q5: How has beta-Parinaric acid been used to investigate biological membranes?
A5: Research has demonstrated the successful incorporation of beta-Parinaric acid into the membranes of living cells, particularly the fatty acid auxotroph E. coli 30E betaox. [] This allows for the study of membrane phase transitions in a more biologically relevant context. By monitoring the fluorescence properties of the incorporated beta-Parinaric acid, researchers can gain insights into the fluidity and phase behavior of bacterial membranes under various conditions and in response to environmental changes. [] This highlights the potential of beta-Parinaric acid as a tool for studying membrane dynamics in living systems.
体外研究产品的免责声明和信息
请注意,BenchChem 上展示的所有文章和产品信息仅供信息参考。 BenchChem 上可购买的产品专为体外研究设计,这些研究在生物体外进行。体外研究,源自拉丁语 "in glass",涉及在受控实验室环境中使用细胞或组织进行的实验。重要的是要注意,这些产品没有被归类为药物或药品,他们没有得到 FDA 的批准,用于预防、治疗或治愈任何医疗状况、疾病或疾病。我们必须强调,将这些产品以任何形式引入人类或动物的身体都是法律严格禁止的。遵守这些指南对确保研究和实验的法律和道德标准的符合性至关重要。