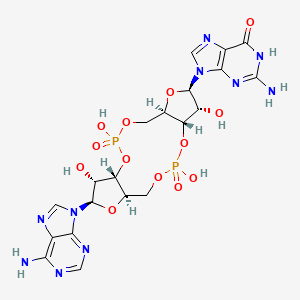
环状二鸟苷酸 (cGAMP)
描述
Cyclic guanosine monophosphate-adenosine monophosphate (cGAMP) is a cyclic dinucleotide that plays a crucial role in the immune response. It is synthesized by the enzyme cyclic GMP-AMP synthase (cGAS) upon detection of cytosolic double-stranded DNA (dsDNA). cGAMP acts as a second messenger, activating the stimulator of interferon genes (STING) pathway, which leads to the production of type I interferons and other pro-inflammatory cytokines .
科学研究应用
cGAMP has a wide range of scientific research applications:
Chemistry: cGAMP is used as a model compound to study the synthesis and degradation of cyclic dinucleotides.
Biology: It is a key molecule in the study of innate immune responses and the cGAS-STING pathway.
Medicine: cGAMP is being explored as a potential therapeutic agent for cancer immunotherapy and antiviral treatments.
作用机制
Mode of Action
cGAMP is synthesized by the DNA sensor cyclic Guanosine Monophosphate-Adenosine Monophosphate synthase (cGAS) in response to the presence of cytosolic double-stranded DNA . Once synthesized, cGAMP binds to and activates STING . This binding induces conformational changes and oligomerization of STING , leading to a series of immune responses .
Pharmacokinetics
It is known that cgamp acts as a second messenger within cells, and it can be easily exported out of the cell, functioning as a soluble extracellular immunotransmitter .
Action Environment
The action of cGAMP can be influenced by various environmental factors. For instance, the presence of double-stranded DNA in the cytoplasm, which can come from invading pathogens or host cells, can activate cGAS and trigger the synthesis of cGAMP . Additionally, the production of cGAMP can be enhanced under certain conditions, such as specific media composition, supplementation of divalent cations, and certain temperatures of protein expression .
生化分析
Biochemical Properties
Cyclic GMP-AMP is involved in various biochemical reactions, primarily through its interaction with the STING protein. Upon binding to double-stranded DNA, cyclic GMP-AMP synthase catalyzes the formation of cyclic GMP-AMP from ATP and GTP. Cyclic GMP-AMP then binds to the STING protein, which is located on the endoplasmic reticulum membrane. This binding induces a conformational change in STING, leading to its activation and subsequent recruitment of downstream signaling molecules such as TBK1 and IRF3. These interactions result in the production of type I interferons and other inflammatory cytokines .
Cellular Effects
Cyclic GMP-AMP has significant effects on various cell types and cellular processes. In immune cells, such as macrophages and dendritic cells, cyclic GMP-AMP activates the STING pathway, leading to the production of type I interferons and pro-inflammatory cytokines. This activation enhances the immune response against viral infections and other pathogens. Additionally, cyclic GMP-AMP influences cell signaling pathways, gene expression, and cellular metabolism. For example, it can induce autophagy, a process that helps to eliminate intracellular pathogens and damaged cellular components .
Molecular Mechanism
The molecular mechanism of cyclic GMP-AMP involves its binding to the STING protein, which triggers a cascade of signaling events. Upon binding to double-stranded DNA, cyclic GMP-AMP synthase produces cyclic GMP-AMP, which then binds to STING. This binding causes a conformational change in STING, leading to its activation. Activated STING recruits and activates TBK1, which in turn phosphorylates IRF3. Phosphorylated IRF3 translocates to the nucleus, where it induces the expression of type I interferons and other cytokines. This signaling cascade is crucial for the antiviral and immune response mediated by cyclic GMP-AMP .
Temporal Effects in Laboratory Settings
In laboratory settings, the effects of cyclic GMP-AMP can vary over time. The stability and degradation of cyclic GMP-AMP are important factors that influence its activity. Studies have shown that cyclic GMP-AMP is relatively stable in vitro, but its degradation can be accelerated by certain enzymes, such as phosphodiesterases. Long-term effects of cyclic GMP-AMP on cellular function have been observed in both in vitro and in vivo studies. For example, prolonged activation of the STING pathway by cyclic GMP-AMP can lead to chronic inflammation and autoimmune diseases .
Dosage Effects in Animal Models
The effects of cyclic GMP-AMP can vary with different dosages in animal models. Low doses of cyclic GMP-AMP can effectively activate the STING pathway and induce an immune response, while high doses can lead to toxic or adverse effects. For example, high doses of cyclic GMP-AMP have been associated with excessive production of type I interferons and pro-inflammatory cytokines, which can result in tissue damage and inflammation. Threshold effects have also been observed, where a minimum concentration of cyclic GMP-AMP is required to activate the STING pathway .
Metabolic Pathways
Cyclic GMP-AMP is involved in several metabolic pathways, including its synthesis by cyclic GMP-AMP synthase and its degradation by phosphodiesterases. The synthesis of cyclic GMP-AMP from ATP and GTP is catalyzed by cyclic GMP-AMP synthase upon recognition of double-stranded DNA. The degradation of cyclic GMP-AMP is mediated by phosphodiesterases, which hydrolyze the cyclic bond, resulting in the formation of linear GMP-AMP. These metabolic pathways are crucial for regulating the levels and activity of cyclic GMP-AMP in cells .
Transport and Distribution
Cyclic GMP-AMP is transported and distributed within cells and tissues through various mechanisms. It can diffuse freely within the cytoplasm and interact with the STING protein on the endoplasmic reticulum membrane. Additionally, cyclic GMP-AMP can be transported between cells through gap junctions or by extracellular vesicles. The distribution of cyclic GMP-AMP within tissues is influenced by its stability and degradation, as well as the presence of transporters and binding proteins that facilitate its movement .
Subcellular Localization
The subcellular localization of cyclic GMP-AMP is primarily in the cytoplasm, where it interacts with the STING protein on the endoplasmic reticulum membrane. Recent studies have shown that cyclic GMP-AMP can also be localized in other subcellular compartments, such as the nucleus and mitochondria. The localization of cyclic GMP-AMP is influenced by targeting signals and post-translational modifications that direct it to specific compartments or organelles. These localization patterns are important for the activity and function of cyclic GMP-AMP in different cellular contexts .
准备方法
Synthetic Routes and Reaction Conditions
The chemical synthesis of cGAMP involves the cyclodimerization of adenosine triphosphate (ATP) and guanosine triphosphate (GTP) catalyzed by cGAS. This process can be carried out in vitro using purified cGAS enzyme and appropriate reaction conditions, including the presence of divalent cations like magnesium or manganese .
Industrial Production Methods
Industrial production of cGAMP can be achieved through recombinant DNA technology. Escherichia coli is commonly used as a host for the expression of recombinant cGAS. The enzyme is then used to catalyze the production of cGAMP from ATP and GTP. This method is advantageous due to its high yield and environmentally friendly nature, as it avoids the use of organic solvents .
化学反应分析
Types of Reactions
cGAMP undergoes various chemical reactions, including hydrolysis and enzymatic degradation. It is hydrolyzed by ectonucleotide pyrophosphatase/phosphodiesterase 1 (ENPP1) to produce guanosine monophosphate (GMP) and adenosine monophosphate (AMP) .
Common Reagents and Conditions
The hydrolysis of cGAMP is typically carried out in aqueous solutions at physiological pH. ENPP1 is the primary enzyme responsible for this reaction, and its activity can be modulated by various inhibitors and activators .
Major Products Formed
The major products formed from the hydrolysis of cGAMP are GMP and AMP. These products are further metabolized by cellular enzymes to participate in various biochemical pathways .
相似化合物的比较
Similar Compounds
Cyclic di-GMP (c-di-GMP): A bacterial second messenger involved in biofilm formation and motility.
Cyclic di-AMP (c-di-AMP): Another bacterial second messenger that regulates cell wall homeostasis and osmoprotection.
Uniqueness of cGAMP
cGAMP is unique among cyclic dinucleotides due to its mixed phosphodiester linkages (2’-5’ and 3’-5’) and its role in the mammalian immune response. Unlike c-di-GMP and c-di-AMP, which are primarily found in bacteria, cGAMP is synthesized by mammalian cells and plays a critical role in antiviral and antitumor immunity .
属性
IUPAC Name |
2-amino-9-[(1S,6R,8R,9R,10S,15R,17R,18R)-17-(6-aminopurin-9-yl)-3,9,12,18-tetrahydroxy-3,12-dioxo-2,4,7,11,13,16-hexaoxa-3λ5,12λ5-diphosphatricyclo[13.3.0.06,10]octadecan-8-yl]-1H-purin-6-one | |
---|---|---|
Source | PubChem | |
URL | https://pubchem.ncbi.nlm.nih.gov | |
Description | Data deposited in or computed by PubChem | |
InChI |
InChI=1S/C20H24N10O13P2/c21-14-8-15(24-3-23-14)29(4-25-8)18-10(31)12-6(40-18)1-38-45(36,37)43-13-7(2-39-44(34,35)42-12)41-19(11(13)32)30-5-26-9-16(30)27-20(22)28-17(9)33/h3-7,10-13,18-19,31-32H,1-2H2,(H,34,35)(H,36,37)(H2,21,23,24)(H3,22,27,28,33)/t6-,7-,10-,11-,12-,13-,18-,19-/m1/s1 | |
Source | PubChem | |
URL | https://pubchem.ncbi.nlm.nih.gov | |
Description | Data deposited in or computed by PubChem | |
InChI Key |
RFCBNSCSPXMEBK-INFSMZHSSA-N | |
Source | PubChem | |
URL | https://pubchem.ncbi.nlm.nih.gov | |
Description | Data deposited in or computed by PubChem | |
Canonical SMILES |
C1C2C(C(C(O2)N3C=NC4=C3N=C(NC4=O)N)O)OP(=O)(OCC5C(C(C(O5)N6C=NC7=C(N=CN=C76)N)O)OP(=O)(O1)O)O | |
Source | PubChem | |
URL | https://pubchem.ncbi.nlm.nih.gov | |
Description | Data deposited in or computed by PubChem | |
Isomeric SMILES |
C1[C@@H]2[C@H]([C@H]([C@@H](O2)N3C=NC4=C3N=C(NC4=O)N)O)OP(=O)(OC[C@@H]5[C@H]([C@H]([C@@H](O5)N6C=NC7=C(N=CN=C76)N)O)OP(=O)(O1)O)O | |
Source | PubChem | |
URL | https://pubchem.ncbi.nlm.nih.gov | |
Description | Data deposited in or computed by PubChem | |
Molecular Formula |
C20H24N10O13P2 | |
Source | PubChem | |
URL | https://pubchem.ncbi.nlm.nih.gov | |
Description | Data deposited in or computed by PubChem | |
DSSTOX Substance ID |
DTXSID401045334 | |
Record name | 3',5'-Cyclic GMP-AMP | |
Source | EPA DSSTox | |
URL | https://comptox.epa.gov/dashboard/DTXSID401045334 | |
Description | DSSTox provides a high quality public chemistry resource for supporting improved predictive toxicology. | |
Molecular Weight |
674.4 g/mol | |
Source | PubChem | |
URL | https://pubchem.ncbi.nlm.nih.gov | |
Description | Data deposited in or computed by PubChem | |
CAS No. |
849214-04-6 | |
Record name | 3',5'-Cyclic GMP-AMP | |
Source | EPA DSSTox | |
URL | https://comptox.epa.gov/dashboard/DTXSID401045334 | |
Description | DSSTox provides a high quality public chemistry resource for supporting improved predictive toxicology. | |
Retrosynthesis Analysis
AI-Powered Synthesis Planning: Our tool employs the Template_relevance Pistachio, Template_relevance Bkms_metabolic, Template_relevance Pistachio_ringbreaker, Template_relevance Reaxys, Template_relevance Reaxys_biocatalysis model, leveraging a vast database of chemical reactions to predict feasible synthetic routes.
One-Step Synthesis Focus: Specifically designed for one-step synthesis, it provides concise and direct routes for your target compounds, streamlining the synthesis process.
Accurate Predictions: Utilizing the extensive PISTACHIO, BKMS_METABOLIC, PISTACHIO_RINGBREAKER, REAXYS, REAXYS_BIOCATALYSIS database, our tool offers high-accuracy predictions, reflecting the latest in chemical research and data.
Strategy Settings
Precursor scoring | Relevance Heuristic |
---|---|
Min. plausibility | 0.01 |
Model | Template_relevance |
Template Set | Pistachio/Bkms_metabolic/Pistachio_ringbreaker/Reaxys/Reaxys_biocatalysis |
Top-N result to add to graph | 6 |
Feasible Synthetic Routes
Q1: How does cGAMP interact with its target and what are the downstream effects?
A: cGAMP acts as a second messenger, primarily by binding to and activating the stimulator of interferon genes (STING) protein located on the endoplasmic reticulum []. This binding triggers a signaling cascade involving TANK-binding kinase 1 (TBK1) and interferon regulatory factor 3 (IRF3), ultimately leading to the production of type I interferons (IFNs) and pro-inflammatory cytokines []. These molecules play crucial roles in antiviral and antitumor immunity.
Q2: Can cGAMP be sensed by cells other than the cell that produced it?
A: Yes, cGAMP can act in both an intracrine and paracrine manner. Research shows that cancer cells release cGAMP into the extracellular space, where it acts as an immunotransmitter []. This extracellular cGAMP can be taken up by neighboring cells, including immune cells, where it activates the STING pathway, triggering antitumor immune responses [, , ].
Q3: What is the molecular formula and weight of cGAMP?
A3: The molecular formula for cGAMP is C20H24N10O14P2. It has a molecular weight of 674.4 g/mol.
Q4: Is there more than one structural isomer of cGAMP?
A: Yes, cGAMP exists in two main isomeric forms: 2′,3′-cGAMP and 3′,3′-cGAMP. The 2′,3′-cGAMP isomer, produced by the mammalian enzyme cGAS, is a potent activator of the STING pathway []. In contrast, the 3′,3′-cGAMP isomer, primarily found in bacteria, exhibits weaker binding affinity to human STING [, , ].
Q5: Is cGAMP stable under physiological conditions?
A: cGAMP is relatively unstable in physiological environments due to the presence of enzymes like ENPP1 that can degrade it [, ].
Q6: How does cGAMP stability impact its potential therapeutic applications?
A: The instability of cGAMP poses a significant challenge for its therapeutic use. Researchers are exploring various strategies to enhance its stability, such as encapsulation in microparticles or nanoparticles, to protect it from degradation and facilitate efficient delivery to target cells [, , ].
Q7: Does cGAMP possess any catalytic properties?
A7: cGAMP itself is not known to have catalytic properties. It functions as a signaling molecule, activating downstream signaling pathways upon binding to its receptor, STING.
Q8: Have computational methods been used to study cGAMP and its interactions?
A: Yes, computational chemistry techniques like molecular dynamics simulations have been employed to investigate the binding interactions of cGAMP with STING [, ]. These simulations provide insights into the structural basis of cGAMP recognition by STING and aid in understanding the differential activation of STING by different CDN isomers.
Q9: How do structural modifications of cGAMP affect its activity and potency?
A: Studies indicate that even subtle modifications to the cGAMP structure, such as changes in the phosphodiester bond configuration, can significantly impact its binding affinity and activation of STING []. For instance, the 2′,3′-cGAMP isomer exhibits greater potency compared to the 3′,3′-cGAMP isomer in activating human STING.
Q10: Are there any known synthetic analogs of cGAMP with improved properties?
A: Yes, researchers have developed synthetic CDN analogs like ADU-S100, a bisphosphosphothioate analog of 2′3′-cGAMP, with enhanced stability and therapeutic potential [, ].
Q11: What are the main challenges in formulating cGAMP for therapeutic use?
A: The major hurdles include its susceptibility to enzymatic degradation, poor cellular uptake due to its hydrophilicity, and rapid clearance from the body [, ].
Q12: What strategies are being explored to improve cGAMP stability and delivery?
A12: Researchers are actively investigating strategies like:
Q13: Are there specific SHE regulations regarding the research and development of cGAMP-based therapies?
A13: While specific regulations for cGAMP are still evolving, general SHE guidelines for handling potent immunomodulatory agents and nanoparticles are applicable. This includes appropriate laboratory safety protocols, risk assessment, waste management, and ethical considerations for preclinical and clinical studies.
Q14: What is known about the absorption, distribution, metabolism, and excretion (ADME) of cGAMP?
A: Research on cGAMP's PK/PD is still ongoing. Due to its instability and rapid degradation by ENPP1, the systemic exposure of cGAMP is limited [, ]. Strategies to enhance its stability and delivery are crucial for improving its PK/PD profile.
Q15: What is the evidence supporting the antitumor activity of cGAMP?
A15: Studies have shown that:
Q16: Are there known mechanisms of resistance to cGAMP-mediated immune activation?
A16: Yes, research has identified several resistance mechanisms:
Q17: What are the potential toxicities associated with cGAMP-based therapies?
A17: As a potent immune stimulator, potential adverse effects of cGAMP may include:
Q18: What are the main challenges in delivering cGAMP specifically to tumor cells?
A18: cGAMP faces challenges like:
Q19: How can nanoparticle-based delivery systems improve cGAMP's therapeutic index?
A19: Nanoparticles offer several advantages:
Q20: Are there any known biomarkers that can predict the efficacy of cGAMP-based therapies?
A20: Research is ongoing to identify predictive biomarkers for cGAMP:
Q21: What analytical methods are commonly used to quantify cGAMP levels?
A21: Sensitive and specific techniques for cGAMP quantification include:
Q22: Is there any information available on the environmental impact and degradation of cGAMP?
A22: Currently, there is limited data available on the environmental fate and potential ecotoxicological effects of cGAMP. Further studies are needed to assess its environmental persistence, bioaccumulation potential, and effects on aquatic and terrestrial organisms.
Q23: How are analytical methods for cGAMP quantification validated?
A23: Validation of analytical methods for cGAMP, like LC-MS/MS or ELISA, involves establishing various performance characteristics, including:
Q24: What quality control measures are crucial during the development and manufacturing of cGAMP-based therapeutics?
A24: Maintaining stringent quality control is essential throughout the entire process:
Q25: Can cGAMP itself elicit an immune response?
A25: While cGAMP is a potent immune activator, its potential immunogenicity is still under investigation. Strategies to modify its structure or formulation might be necessary to modulate its immunogenicity and minimize potential adverse effects.
Q26: Is there information available on cGAMP's interactions with drug transporters, metabolizing enzymes, and its biocompatibility?
A26: Research in these areas is ongoing. Understanding cGAMP's interactions with drug transporters and metabolizing enzymes is crucial for predicting its pharmacokinetic behavior and potential drug-drug interactions. Investigating its biocompatibility and biodegradability is essential for ensuring the safety and efficacy of cGAMP-based therapies.
Q27: Are there any alternatives to cGAMP as STING agonists for therapeutic applications?
A27: Yes, several synthetic CDN analogs and non-CDN STING agonists are being explored as alternatives to cGAMP, each with its advantages and limitations. Examples include:
Q28: What strategies are being considered for the recycling and waste management of cGAMP and related materials?
A28: As with many emerging pharmaceuticals, specific guidelines for recycling and waste management of cGAMP are still under development. Strategies might involve:
Q29: What research infrastructure and resources are crucial for advancing cGAMP-based therapies?
A29: Key resources include:
Q30: What are some key milestones in the research and development of cGAMP?
A30: Significant discoveries include:
- Identification of cGAMP as a Second Messenger: The discovery of cGAMP as a second messenger produced by the DNA sensor cGAS revolutionized our understanding of innate immune signaling [].
- Recognition of cGAMP's Role as an Immunotransmitter: The finding that cGAMP can be secreted by cancer cells and act as an immunotransmitter, activating the STING pathway in neighboring cells, opened new avenues for cancer immunotherapy [, , ].
体外研究产品的免责声明和信息
请注意,BenchChem 上展示的所有文章和产品信息仅供信息参考。 BenchChem 上可购买的产品专为体外研究设计,这些研究在生物体外进行。体外研究,源自拉丁语 "in glass",涉及在受控实验室环境中使用细胞或组织进行的实验。重要的是要注意,这些产品没有被归类为药物或药品,他们没有得到 FDA 的批准,用于预防、治疗或治愈任何医疗状况、疾病或疾病。我们必须强调,将这些产品以任何形式引入人类或动物的身体都是法律严格禁止的。遵守这些指南对确保研究和实验的法律和道德标准的符合性至关重要。