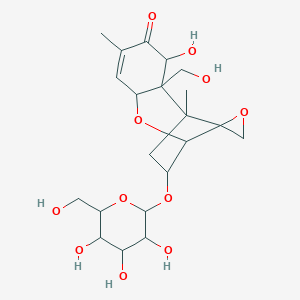
脱氧雪腐镰刀菌烯醇-3-葡萄糖苷
描述
Certan Vial
Deoxynivalenol 3-glucoside solution is the major metabolite of deoxynivalenol (DON) and a modified mycotoxin. The conjugation of deoxynivalenol (DON) with glucose formed by the metabolism of plants results in the formation of deoxynivalenol-3-glucoside.
Deoxynivalenol 3-glucoside belongs to the class of organic compounds known as trichothecenes. These are sesquiterpene mycotoxins structurally characterized by the presence of an epoxide ring and a benzopyran derivative with a variant number of hydroxyl, acetyl, or other substituents. The most important structural features causing the biological activities of trichothecenes are the 12, 13-epoxy ring, the presence of hydroxyl or acetyl groups at appropriate positions on the trichothecene nucleus and the structure and position of the side-chain. Deoxynivalenol 3-glucoside is soluble (in water) and a very weakly acidic compound (based on its pKa). Within the cell, deoxynivalenol 3-glucoside is primarily located in the cytoplasm. Outside of the human body, deoxynivalenol 3-glucoside can be found in cereals and cereal products and fats and oils. This makes deoxynivalenol 3-glucoside a potential biomarker for the consumption of these food products.
科学研究应用
Toxicological Relevance and Metabolism
D3G is often referred to as a "masked" mycotoxin because it can be converted back into DON in the digestive tract. Studies indicate that D3G is hydrolyzed to DON during digestion, raising concerns about its toxicological relevance.
- Metabolism in Animals : Research involving rats showed that D3G is metabolized by gut microbiota, with only a small fraction excreted unchanged in urine. Most of the administered D3G was recovered as DON and other metabolites in feces, indicating that while D3G itself is less toxic than DON, it can still contribute to overall DON exposure in humans and animals .
- Human Studies : A human intervention study demonstrated that D3G is rapidly absorbed and metabolized. Approximately 58% of administered D3G was recovered in urine within 24 hours, predominantly as glucuronide conjugates . This highlights the importance of monitoring both DON and its glucoside form in dietary assessments.
Food Safety and Regulatory Implications
The presence of D3G in food products has significant implications for food safety regulations.
- Occurrence in Cereals : D3G has been detected in various cereal grains, including wheat and maize, often as part of a defense mechanism against Fusarium infection. Its levels can vary based on environmental conditions and agricultural practices .
- Regulatory Challenges : Current food safety regulations primarily focus on DON levels without considering D3G. However, understanding the dynamics between these compounds is crucial for accurate risk assessment and regulatory frameworks .
Analytical Methods for Detection
The detection and quantification of D3G are critical for both research and regulatory purposes.
- Analytical Techniques : Advanced techniques such as liquid chromatography coupled with mass spectrometry (LC-MS/MS) have been employed to analyze D3G levels in food samples. These methods provide reliable data on the occurrence of both DON and D3G in cereals .
- Case Studies : Various studies have reported the concentrations of D3G in different wheat varieties under varying conditions. For example, a study conducted on hard red spring wheat inoculated with Fusarium graminearum found a positive correlation between DON and D3G levels, suggesting that higher DON levels lead to increased D3G formation as part of the plant's detoxification response .
Plant Defense Mechanisms
D3G plays a significant role in the plant's defense against fungal pathogens.
- Detoxification Process : The formation of D3G occurs through glycosylation, which serves as a detoxification strategy for plants exposed to DON-producing fungi. This process helps mitigate the harmful effects of mycotoxins on plant health .
- Research Implications : Understanding how plants produce D3G can inform breeding programs aimed at enhancing resistance to Fusarium infections, thereby reducing both DON and D3G levels in crops .
作用机制
Target of Action
Deoxynivalenol-3-glucoside (D3G) is a modified mycotoxin formed by the metabolism of plants through the conjugation of deoxynivalenol (DON) with glucose . The primary target of D3G, like DON, is the ribosome . It binds to the 60S subunit of the ribosome , which plays a crucial role in protein synthesis.
Mode of Action
D3G is hydrolyzed to DON during digestion . , but also activates intracellular protein kinases that mediate selective gene expression and apoptosis. This disruption of macromolecule synthesis, cell signaling, differentiation, proliferation, and death is a key aspect of its mode of action .
Biochemical Pathways
The biochemical pathways affected by DON and, by extension, D3G include the TNF-α-induced NF-κB signaling pathway . DON induces the TNF-α-converting enzyme-dependent ectodomain shedding of TNF-receptor 1 via the activation of ERK and p38 MAP kinase, thereby inhibiting the TNF-α-induced NF-κB signaling pathway .
Pharmacokinetics
D3G is resistant to acids and enzymes expressed by humans . It is partly cleaved by cellulase and cellobiase, and several intestinal bacteria liberate don from d3g . This suggests that the bioavailability of D3G and its impact on health may be influenced by the gut microbiota. In addition, age-related differences in the toxicokinetic processes of DON and D3G have been observed .
Result of Action
The molecular and cellular effects of D3G’s action are primarily due to its hydrolysis to DON during digestion . DON causes emesis, anorexia, growth retardation, immunotoxicity, and impaired reproduction and development . At the cellular level, DON induces ribotoxic stress, disrupting macromolecule synthesis, cell signaling, differentiation, proliferation, and death .
Action Environment
The production of DON and D3G can be triggered by diverse environmental and cellular signals . Many environmental factors, such as humidity and intensive rainfall, affect DON levels during the infection process . Moreover, thermal food processing can influence the stability of DON and the formation of degradation/conversion products .
生化分析
Biochemical Properties
Deoxynivalenol 3-glucoside is formed through the enzymatic interaction of DON with glucose . It interacts with various enzymes, proteins, and other biomolecules in the body. For instance, it has been suggested that DON-3G can be enzymatically hydrolyzed by intestinal bacteria . The nature of these interactions is complex and involves various biochemical reactions.
Cellular Effects
Deoxynivalenol 3-glucoside, like its parent compound DON, can have significant effects on various types of cells and cellular processes . It has been suggested that DON-3G can influence cell function, including impacts on cell signaling pathways, gene expression, and cellular metabolism . The bioavailability of DON-3G is probably low compared to that of DON, as human Caco-2 cells do not absorb DON-3G .
Molecular Mechanism
It is known that DON, the parent compound of DON-3G, binds to the ribosome, induces a ribotoxic stress leading to the activation of MAP kinases, cellular cell-cycle arrest and apoptosis . It is plausible that DON-3G may exert similar effects at the molecular level once it is converted back to DON during digestion .
Temporal Effects in Laboratory Settings
In laboratory settings, the effects of Deoxynivalenol 3-glucoside can change over time. For instance, it has been found that DON-3G is resistant to 0.2M hydrochloric acid for at least 24 hours at 37°C, suggesting that it will not be hydrolyzed in the stomach of mammals . This indicates that the product’s stability and long-term effects on cellular function can vary depending on the conditions of the experiment.
Dosage Effects in Animal Models
The effects of Deoxynivalenol 3-glucoside can vary with different dosages in animal models. For instance, in a study on mice, it was found that oral administration of DON and DON-3G at doses from 2.5 to 10 mg/kg body weight induced anorectic responses
Metabolic Pathways
Deoxynivalenol 3-glucoside is involved in metabolic pathways that involve the conversion of DON to DON-3G through the glycosylation of DON . This process is connected with glycosyltransferases
Transport and Distribution
Deoxynivalenol 3-glucoside, like DON, is fast-absorbed and widely distributed in multiple organs. It is first enriched in the plasma, liver, and kidney and subsequently accumulates in the intestine
Subcellular Localization
A related study has shown that a specific UDP-glycosyltransferase gene (TaUGT5), which is involved in the detoxification of DON, was localized on the plasma membrane of tobacco leaf epidermal cells
生物活性
Deoxynivalenol-3-glucoside (DON-3G) is a plant metabolite derived from deoxynivalenol (DON), a mycotoxin produced by various species of the Fusarium fungi. This compound has garnered attention due to its prevalence in contaminated cereals and its potential effects on human and animal health. This article delves into the biological activity of DON-3G, including its metabolism, toxicological relevance, and implications for health based on recent research findings.
Overview of Deoxynivalenol and Its Glucoside
Deoxynivalenol (DON) is known for its acute toxicity, primarily causing anorectic (appetite-suppressing) and emetic (vomiting-inducing) effects in various species, including humans. DON-3G, on the other hand, is considered a "masked" mycotoxin that may mitigate some of the toxic effects associated with DON due to its lower bioavailability and toxicity.
Metabolism and Bioavailability
Research indicates that DON-3G is readily hydrolyzed to DON during digestion. A study involving Sprague-Dawley rats demonstrated that after administration of DON-3G, only about 3.7% was found in urine as analyzed metabolites, compared to 14.9% for DON itself, suggesting that DON-3G has significantly lower bioavailability . The majority of administered DON-3G was recovered as DON in feces, indicating that it undergoes extensive metabolism by gut microbiota before excretion .
Table 1: Metabolism of DON and DON-3G in Rats
Compound | Dose (mg/kg) | Urinary Recovery (%) | Fecal Recovery (%) |
---|---|---|---|
Deoxynivalenol (DON) | 2.0 | 14.9 ± 5.0 | Not specified |
Deoxynivalenol-3-glucoside (DON-3G) | 3.1 | 3.7 ± 0.7 | Predominantly as DON |
Toxicological Relevance
In terms of toxicological impact, studies suggest that DON-3G is considerably less toxic than its parent compound. For instance, a study involving human volunteers showed that both DON and DON-3G were rapidly absorbed and metabolized; however, the recovery of DON from urine was higher than that of DON-3G . Specifically, 58% of administered DON-3G was recovered in urine within 24 hours, indicating its rapid metabolism but also highlighting its lower potential for toxicity compared to free DON .
Case Study: Anorectic Response in Mice
A comparative study assessed the anorectic responses induced by DON, DON-3G, and synthetic derivatives in mice. Results indicated that while all compounds elicited food refusal behaviors, the potency was markedly lower for DON-3G compared to DON. The anorectic effects lasted up to 6 hours for DON-3G versus up to 16 hours for DON . This finding underscores the reduced biological activity of DON-3G relative to its parent compound.
Implications for Human Health
The implications of these findings are significant for food safety and regulatory standards concerning mycotoxin exposure. Given that DON is a well-established public health concern due to its acute toxicity and chronic health effects, understanding the role of its metabolites like DON-3G is crucial. Regulatory agencies have yet to incorporate specific limits for masked mycotoxins like DON-3G due to insufficient data on their toxicological profiles.
属性
IUPAC Name |
(1R,2R,3S,7R,9R,10R,12S)-3-hydroxy-2-(hydroxymethyl)-1,5-dimethyl-10-[(2R,3R,4S,5S,6R)-3,4,5-trihydroxy-6-(hydroxymethyl)oxan-2-yl]oxyspiro[8-oxatricyclo[7.2.1.02,7]dodec-5-ene-12,2'-oxirane]-4-one | |
---|---|---|
Source | PubChem | |
URL | https://pubchem.ncbi.nlm.nih.gov | |
Description | Data deposited in or computed by PubChem | |
InChI |
InChI=1S/C21H30O11/c1-8-3-11-20(6-23,16(28)12(8)24)19(2)4-9(17(32-11)21(19)7-29-21)30-18-15(27)14(26)13(25)10(5-22)31-18/h3,9-11,13-18,22-23,25-28H,4-7H2,1-2H3/t9-,10-,11-,13-,14+,15-,16-,17-,18-,19-,20-,21+/m1/s1 | |
Source | PubChem | |
URL | https://pubchem.ncbi.nlm.nih.gov | |
Description | Data deposited in or computed by PubChem | |
InChI Key |
PUMXWMGECQIOGB-SMSDQXDJSA-N | |
Source | PubChem | |
URL | https://pubchem.ncbi.nlm.nih.gov | |
Description | Data deposited in or computed by PubChem | |
Canonical SMILES |
CC1=CC2C(C(C1=O)O)(C3(CC(C(C34CO4)O2)OC5C(C(C(C(O5)CO)O)O)O)C)CO | |
Source | PubChem | |
URL | https://pubchem.ncbi.nlm.nih.gov | |
Description | Data deposited in or computed by PubChem | |
Isomeric SMILES |
CC1=C[C@@H]2[C@]([C@@H](C1=O)O)([C@]3(C[C@H]([C@H]([C@@]34CO4)O2)O[C@H]5[C@@H]([C@H]([C@@H]([C@H](O5)CO)O)O)O)C)CO | |
Source | PubChem | |
URL | https://pubchem.ncbi.nlm.nih.gov | |
Description | Data deposited in or computed by PubChem | |
Molecular Formula |
C21H30O11 | |
Source | PubChem | |
URL | https://pubchem.ncbi.nlm.nih.gov | |
Description | Data deposited in or computed by PubChem | |
DSSTOX Substance ID |
DTXSID10891865 | |
Record name | Deoxynivalenol-3-O-glucoside | |
Source | EPA DSSTox | |
URL | https://comptox.epa.gov/dashboard/DTXSID10891865 | |
Description | DSSTox provides a high quality public chemistry resource for supporting improved predictive toxicology. | |
Molecular Weight |
458.5 g/mol | |
Source | PubChem | |
URL | https://pubchem.ncbi.nlm.nih.gov | |
Description | Data deposited in or computed by PubChem | |
CAS No. |
131180-21-7 | |
Record name | Deoxynivalenol 3-glucoside | |
Source | CAS Common Chemistry | |
URL | https://commonchemistry.cas.org/detail?cas_rn=131180-21-7 | |
Description | CAS Common Chemistry is an open community resource for accessing chemical information. Nearly 500,000 chemical substances from CAS REGISTRY cover areas of community interest, including common and frequently regulated chemicals, and those relevant to high school and undergraduate chemistry classes. This chemical information, curated by our expert scientists, is provided in alignment with our mission as a division of the American Chemical Society. | |
Explanation | The data from CAS Common Chemistry is provided under a CC-BY-NC 4.0 license, unless otherwise stated. | |
Record name | Deoxynivalenol-3-O-glucoside | |
Source | ChemIDplus | |
URL | https://pubchem.ncbi.nlm.nih.gov/substance/?source=chemidplus&sourceid=0131180217 | |
Description | ChemIDplus is a free, web search system that provides access to the structure and nomenclature authority files used for the identification of chemical substances cited in National Library of Medicine (NLM) databases, including the TOXNET system. | |
Record name | Deoxynivalenol-3-O-glucoside | |
Source | EPA DSSTox | |
URL | https://comptox.epa.gov/dashboard/DTXSID10891865 | |
Description | DSSTox provides a high quality public chemistry resource for supporting improved predictive toxicology. | |
Record name | Deoxynivalenol 3-glucoside | |
Source | European Chemicals Agency (ECHA) | |
URL | https://echa.europa.eu/information-on-chemicals | |
Description | The European Chemicals Agency (ECHA) is an agency of the European Union which is the driving force among regulatory authorities in implementing the EU's groundbreaking chemicals legislation for the benefit of human health and the environment as well as for innovation and competitiveness. | |
Explanation | Use of the information, documents and data from the ECHA website is subject to the terms and conditions of this Legal Notice, and subject to other binding limitations provided for under applicable law, the information, documents and data made available on the ECHA website may be reproduced, distributed and/or used, totally or in part, for non-commercial purposes provided that ECHA is acknowledged as the source: "Source: European Chemicals Agency, http://echa.europa.eu/". Such acknowledgement must be included in each copy of the material. ECHA permits and encourages organisations and individuals to create links to the ECHA website under the following cumulative conditions: Links can only be made to webpages that provide a link to the Legal Notice page. | |
Record name | DEOXYNIVALENOL 3-GLUCOSIDE | |
Source | FDA Global Substance Registration System (GSRS) | |
URL | https://gsrs.ncats.nih.gov/ginas/app/beta/substances/HKI01R84M9 | |
Description | The FDA Global Substance Registration System (GSRS) enables the efficient and accurate exchange of information on what substances are in regulated products. Instead of relying on names, which vary across regulatory domains, countries, and regions, the GSRS knowledge base makes it possible for substances to be defined by standardized, scientific descriptions. | |
Explanation | Unless otherwise noted, the contents of the FDA website (www.fda.gov), both text and graphics, are not copyrighted. They are in the public domain and may be republished, reprinted and otherwise used freely by anyone without the need to obtain permission from FDA. Credit to the U.S. Food and Drug Administration as the source is appreciated but not required. | |
Retrosynthesis Analysis
AI-Powered Synthesis Planning: Our tool employs the Template_relevance Pistachio, Template_relevance Bkms_metabolic, Template_relevance Pistachio_ringbreaker, Template_relevance Reaxys, Template_relevance Reaxys_biocatalysis model, leveraging a vast database of chemical reactions to predict feasible synthetic routes.
One-Step Synthesis Focus: Specifically designed for one-step synthesis, it provides concise and direct routes for your target compounds, streamlining the synthesis process.
Accurate Predictions: Utilizing the extensive PISTACHIO, BKMS_METABOLIC, PISTACHIO_RINGBREAKER, REAXYS, REAXYS_BIOCATALYSIS database, our tool offers high-accuracy predictions, reflecting the latest in chemical research and data.
Strategy Settings
Precursor scoring | Relevance Heuristic |
---|---|
Min. plausibility | 0.01 |
Model | Template_relevance |
Template Set | Pistachio/Bkms_metabolic/Pistachio_ringbreaker/Reaxys/Reaxys_biocatalysis |
Top-N result to add to graph | 6 |
Feasible Synthetic Routes
ANone: DON-3-glucoside is formed as a plant defense mechanism against DON. When Fusarium fungi infect crops like wheat, barley, and maize, the plants can convert DON into DON-3-glucoside through glycosylation. This process involves attaching a glucose molecule to DON, effectively detoxifying it and reducing its toxicity to the plant. [, , , , , ]
ANone: DON-3-glucoside is primarily found in cereal grains and cereal-based products. This includes wheat, barley, maize, malt, beer, bread, and various processed foods derived from these grains. Its presence has been reported in samples collected globally, highlighting its widespread occurrence as a food contaminant. [, , , , , , , ]
ANone: Concentrations of DON-3-glucoside in contaminated products vary depending on factors like the type of cereal, environmental conditions, and fungal strain. Studies have reported levels ranging from µg/kg to mg/kg levels in both raw and processed products, with some exceeding the regulatory limits set for DON. [, , , , , , ]
ANone: DON-3-glucoside is considered less toxic than DON due to its modified structure. In vitro and in vivo studies have shown that it exhibits significantly lower toxicity in various models, including microbial cells, porcine intestinal cells, and piglets. [, , ]
ANone: DON-3-glucoside is relatively stable in the upper digestive tract but can be hydrolyzed by the gut microbiota in the distal small and large intestine. This hydrolysis process removes the glucose molecule, converting DON-3-glucoside back into the more toxic DON, which can then be absorbed into the bloodstream. [, , ]
ANone: While DON-3-glucoside itself is less toxic, its potential to convert back to DON in the gut raises concerns about its contribution to overall DON exposure. This "masking" effect makes accurate risk assessment challenging, as routine analytical methods often fail to detect DON-3-glucoside. [, , , , ]
ANone: DON-3-glucoside analysis typically involves chromatographic techniques coupled with mass spectrometry, such as LC-MS/MS (liquid chromatography-tandem mass spectrometry). These methods offer high sensitivity and selectivity, allowing for accurate quantification even at low concentrations. [, , , , ]
ANone: Accurate analysis of DON-3-glucoside can be challenging due to factors like matrix interference, the lack of commercially available standards, and the need for specific extraction and purification procedures. The development of reliable and standardized methods is crucial for monitoring its occurrence in food and feed. [, , , ]
ANone: Strategies to reduce DON-3-glucoside levels focus on preventing Fusarium infection and minimizing DON production in the first place. This includes good agricultural practices, resistant cultivars, and appropriate fungicide treatments. [, , , , ]
ANone: Some food processing methods, like milling and baking, can partially reduce DON-3-glucoside levels, but their effectiveness varies depending on the specific process and conditions. Research suggests that these processes can also lead to the formation of other DON derivatives. [, , , ]
ANone: Further research is needed to:
体外研究产品的免责声明和信息
请注意,BenchChem 上展示的所有文章和产品信息仅供信息参考。 BenchChem 上可购买的产品专为体外研究设计,这些研究在生物体外进行。体外研究,源自拉丁语 "in glass",涉及在受控实验室环境中使用细胞或组织进行的实验。重要的是要注意,这些产品没有被归类为药物或药品,他们没有得到 FDA 的批准,用于预防、治疗或治愈任何医疗状况、疾病或疾病。我们必须强调,将这些产品以任何形式引入人类或动物的身体都是法律严格禁止的。遵守这些指南对确保研究和实验的法律和道德标准的符合性至关重要。