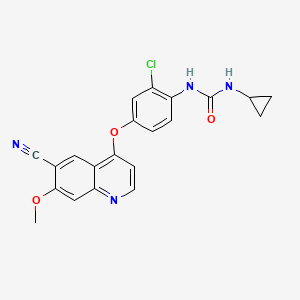
仑伐替尼杂质 8
描述
Lenvatinib Impurity 8, also known as 4-Chloro-7-methoxyquinoline-6-carboxamide, is an impurity of Lenvatinib . Lenvatinib is an orally active inhibitor of multiple receptor tyrosine kinases including VEGF, FGF, and SCF receptors .
Molecular Structure Analysis
The molecular formula of Lenvatinib Impurity 8 is C11H9ClN2O2 . It is structurally represented as 4-Chloro-7-methoxyquinoline-6-carboxamide .Chemical Reactions Analysis
Lenvatinib, the parent compound of Lenvatinib Impurity 8, is absorbed rapidly following oral administration and is metabolized extensively prior to excretion . This metabolism is mediated by multiple pathways, and several metabolites of Lenvatinib have been identified .科学研究应用
药代动力学研究
仑伐替尼是一种用于治疗甲状腺癌的有效药物,它是一种酪氨酸激酶抑制剂。研究的一个重要领域是大鼠血浆中仑伐替尼和相关杂质的开发和验证。Talari 等人 (2022 年) 的一项研究重点是使用 LC-MS/MS 对大鼠血浆中的仑伐替尼及其杂质进行生物分析系统验证和药代动力学分析。这项研究对于了解仑伐替尼及其杂质在生物系统中的药代动力学至关重要 (Talari 等,2022 年).
肿瘤微环境的影响
Kato 等人 (2019 年) 的一项研究调查了仑伐替尼在肿瘤微环境中的免疫调节活性。他们发现仑伐替尼通过减少肿瘤相关巨噬细胞 (TAM) 来调节肿瘤环境中的癌症免疫,并且当与 PD-1 阻断剂联合使用时,通过干扰素信号通路显示出增强的抗肿瘤活性 (Kato 等,2019 年).
代谢途径分析
Inoue 等人 (2014 年) 探索了由醛氧化酶介导的仑伐替尼的氧化代谢途径。这项研究对于了解仑伐替尼在体内的代谢至关重要,并可以为优化其治疗效果和最大限度地减少不良反应提供见解 (Inoue 等,2014 年).
药物监测和定量
开发灵敏、快速且经济高效的方法来定量人血浆中的仑伐替尼对于治疗性药物监测至关重要。Zanchetta 等人 (2021 年) 为此开发了一种 LC-MS/MS 方法,这对于优化癌症治疗的药物剂量中的精准医疗至关重要 (Zanchetta 等,2021 年).
作用机制
Target of Action
Mode of Action
Biochemical Pathways
The primary pathway of the anticancer effect of Lenvatinib is the RAS/RAF/MEK/ERK pathway . Overexpression of FGFR and activation of RAS/RAF/ERK and PI3K/AKT/mTOR signaling contribute to resistance to Lenvatinib . Lenvatinib is unable to completely block the VEGFR-2 and FGFR1 receptors and downstream signaling pathways, which lead to resistance .
Pharmacokinetics
Following oral administration, Lenvatinib is absorbed rapidly and is metabolized extensively prior to excretion . This metabolism is mediated by multiple pathways, and several metabolites of Lenvatinib have been identified . Exposure to Lenvatinib is increased in patients with severe hepatic impairment, indicating that dose reduction must be considered for those patients .
Result of Action
Lenvatinib promotes apoptosis, suppresses angiogenesis, inhibits tumor cell proliferation, and modulates the immune response . In multiple cancer types, Lenvatinib has presented manageable safety and is currently approved as an effective first-line therapy . Resistance to lenvatinib emerges soon after initial treatment, limiting the clinical benefits of lenvatinib .
Action Environment
The action environment of Lenvatinib is influenced by various factors. For instance, the expression of YRDC in hepatocarcinoma cells can modulate the sensitivity of Lenvatinib . Lenvatinib inhibits the expression of YRDC in a time-dependent manner, which may aggravate resistance to Lenvatinib in hepatocarcinoma cells . Furthermore, the tRNA with low t6A modification level reduces the translation of the KRAS in the in vitro translation system .
属性
IUPAC Name |
1-[2-chloro-4-(6-cyano-7-methoxyquinolin-4-yl)oxyphenyl]-3-cyclopropylurea | |
---|---|---|
Source | PubChem | |
URL | https://pubchem.ncbi.nlm.nih.gov | |
Description | Data deposited in or computed by PubChem | |
InChI |
InChI=1S/C21H17ClN4O3/c1-28-20-10-18-15(8-12(20)11-23)19(6-7-24-18)29-14-4-5-17(16(22)9-14)26-21(27)25-13-2-3-13/h4-10,13H,2-3H2,1H3,(H2,25,26,27) | |
Source | PubChem | |
URL | https://pubchem.ncbi.nlm.nih.gov | |
Description | Data deposited in or computed by PubChem | |
InChI Key |
CKIBVUCMZIZNRC-UHFFFAOYSA-N | |
Source | PubChem | |
URL | https://pubchem.ncbi.nlm.nih.gov | |
Description | Data deposited in or computed by PubChem | |
Canonical SMILES |
COC1=CC2=NC=CC(=C2C=C1C#N)OC3=CC(=C(C=C3)NC(=O)NC4CC4)Cl | |
Source | PubChem | |
URL | https://pubchem.ncbi.nlm.nih.gov | |
Description | Data deposited in or computed by PubChem | |
Molecular Formula |
C21H17ClN4O3 | |
Source | PubChem | |
URL | https://pubchem.ncbi.nlm.nih.gov | |
Description | Data deposited in or computed by PubChem | |
Molecular Weight |
408.8 g/mol | |
Source | PubChem | |
URL | https://pubchem.ncbi.nlm.nih.gov | |
Description | Data deposited in or computed by PubChem | |
Retrosynthesis Analysis
AI-Powered Synthesis Planning: Our tool employs the Template_relevance Pistachio, Template_relevance Bkms_metabolic, Template_relevance Pistachio_ringbreaker, Template_relevance Reaxys, Template_relevance Reaxys_biocatalysis model, leveraging a vast database of chemical reactions to predict feasible synthetic routes.
One-Step Synthesis Focus: Specifically designed for one-step synthesis, it provides concise and direct routes for your target compounds, streamlining the synthesis process.
Accurate Predictions: Utilizing the extensive PISTACHIO, BKMS_METABOLIC, PISTACHIO_RINGBREAKER, REAXYS, REAXYS_BIOCATALYSIS database, our tool offers high-accuracy predictions, reflecting the latest in chemical research and data.
Strategy Settings
Precursor scoring | Relevance Heuristic |
---|---|
Min. plausibility | 0.01 |
Model | Template_relevance |
Template Set | Pistachio/Bkms_metabolic/Pistachio_ringbreaker/Reaxys/Reaxys_biocatalysis |
Top-N result to add to graph | 6 |
Feasible Synthetic Routes
体外研究产品的免责声明和信息
请注意,BenchChem 上展示的所有文章和产品信息仅供信息参考。 BenchChem 上可购买的产品专为体外研究设计,这些研究在生物体外进行。体外研究,源自拉丁语 "in glass",涉及在受控实验室环境中使用细胞或组织进行的实验。重要的是要注意,这些产品没有被归类为药物或药品,他们没有得到 FDA 的批准,用于预防、治疗或治愈任何医疗状况、疾病或疾病。我们必须强调,将这些产品以任何形式引入人类或动物的身体都是法律严格禁止的。遵守这些指南对确保研究和实验的法律和道德标准的符合性至关重要。