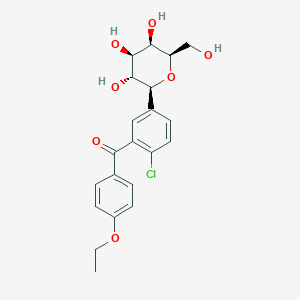
Dapagliflozin Keto Impurity
描述
Dapagliflozin Keto Impurity is a byproduct formed during the synthesis of dapagliflozin, a sodium-glucose cotransporter 2 (SGLT2) inhibitor used in the treatment of type 2 diabetes mellitus. This impurity is significant in the pharmaceutical industry as it can affect the efficacy and safety of the final drug product. Understanding the properties, synthesis, and reactions of this compound is crucial for ensuring the quality and safety of dapagliflozin.
作用机制
Target of Action
Dapagliflozin Keto Impurity primarily targets the sodium-glucose cotransporter 2 (SGLT2) . This transporter is located in the proximal renal tubules and plays a crucial role in reabsorbing filtered glucose from the tubular lumen back into the bloodstream .
Mode of Action
This compound acts by inhibiting the SGLT2 transporter . This inhibition reduces the reabsorption of filtered glucose, leading to an increase in glucose excretion through urine . As a result, blood glucose levels are lowered .
Biochemical Pathways
The action of this compound affects several biochemical pathways. Primarily, it influences the glycolysis/gluconeogenesis and pentose phosphate pathway . By increasing glucose excretion, it reduces glucose availability for these pathways, potentially altering their activity and downstream effects .
Pharmacokinetics
This compound is rapidly and extensively absorbed from the gastrointestinal tract, with an oral bioavailability of 78% . It undergoes extensive metabolism and transforms into metabolites in humans . These pharmacokinetic properties impact the compound’s bioavailability and effectiveness .
Result of Action
The primary molecular effect of this compound’s action is the reduction of blood glucose levels . On a cellular level, this can lead to improvements in insulin sensitivity and β-cell function . Clinically, this results in dose-related reductions in glycosylated hemoglobin (HbA1c), a marker of long-term blood glucose control .
Action Environment
The action, efficacy, and stability of this compound can be influenced by various environmental factors. For instance, the presence of certain impurities in the final product could potentially affect its effectiveness . Additionally, factors such as pH and temperature could influence the stability of the compound and its degradation pathway .
生化分析
Biochemical Properties
Dapagliflozin Keto Impurity plays a role in various biochemical reactions. It interacts with several enzymes, proteins, and other biomolecules. For instance, it has been observed to interact with sodium-glucose co-transporter 2, influencing glucose metabolism. The nature of these interactions involves binding to the active sites of enzymes, thereby inhibiting or modifying their activity. This interaction can lead to changes in the metabolic pathways and overall biochemical environment .
Cellular Effects
This compound affects various types of cells and cellular processes. It influences cell function by altering cell signaling pathways, gene expression, and cellular metabolism. For example, it has been shown to affect the expression of genes involved in glucose metabolism and insulin signaling. Additionally, it can impact cellular metabolism by modifying the activity of key metabolic enzymes, leading to changes in the levels of metabolites and energy production .
Molecular Mechanism
The molecular mechanism of action of this compound involves several key processes. It exerts its effects by binding to specific biomolecules, such as enzymes and receptors, leading to their inhibition or activation. This binding can result in changes in gene expression and enzyme activity, ultimately affecting cellular function. For instance, the impurity can inhibit the activity of sodium-glucose co-transporter 2, leading to reduced glucose reabsorption and increased glucose excretion .
Temporal Effects in Laboratory Settings
In laboratory settings, the effects of this compound can change over time. Studies have shown that the stability and degradation of the impurity can influence its long-term effects on cellular function. For example, prolonged exposure to the impurity can lead to sustained changes in gene expression and enzyme activity, resulting in long-term alterations in cellular metabolism and function .
Dosage Effects in Animal Models
The effects of this compound vary with different dosages in animal models. At lower doses, the impurity may have minimal effects on cellular function, while higher doses can lead to significant changes in metabolic pathways and cellular processes. Toxic or adverse effects have been observed at high doses, including alterations in glucose metabolism and insulin signaling .
Metabolic Pathways
This compound is involved in several metabolic pathways. It interacts with enzymes and cofactors involved in glucose metabolism, leading to changes in metabolic flux and metabolite levels. For instance, the impurity can inhibit the activity of key enzymes in the glycolysis and gluconeogenesis pathways, resulting in altered glucose levels and energy production .
Transport and Distribution
The transport and distribution of this compound within cells and tissues involve several transporters and binding proteins. The impurity can be transported across cell membranes by specific transporters, leading to its accumulation in certain tissues. This localization can affect its activity and function, influencing cellular processes and metabolic pathways .
Subcellular Localization
This compound is localized in specific subcellular compartments, which can affect its activity and function. Targeting signals and post-translational modifications direct the impurity to specific organelles, such as the mitochondria or endoplasmic reticulum. This localization can influence its interactions with other biomolecules and its overall impact on cellular function .
准备方法
The preparation of dapagliflozin keto impurity involves several synthetic routes and reaction conditions. One common method involves the use of 5-Bromo-2-chlorobenzoic acid and 4-Bromo-1-chloro-2-(4-ethoxybenzyl) benzene as starting materials . These reagents undergo a series of reactions, including condensation and cyclization, to form the desired impurity. The reaction mass is then cooled to ambient temperature, and the insolubles are removed by celite bed filtration . Industrial production methods often involve similar synthetic routes but on a larger scale, with optimized reaction conditions to ensure high yield and purity.
化学反应分析
Dapagliflozin keto impurity undergoes various chemical reactions, including oxidation, reduction, and substitution. Common reagents used in these reactions include trifluoroacetic acid, acetonitrile, and potassium bicarbonate . For example, oxidation reactions can lead to the formation of benzylic hydroxy dapagliflozin, while reduction reactions can produce desethyl dapagliflozin . Substitution reactions often involve the replacement of functional groups, leading to the formation of different metabolites. The major products formed from these reactions include benzylic hydroxy dapagliflozin, oxo dapagliflozin, and desethyl dapagliflozin .
科学研究应用
Dapagliflozin keto impurity has several scientific research applications. In chemistry, it is used to study the stability and degradation pathways of dapagliflozin . In biology, it helps in understanding the metabolism and biotransformation of dapagliflozin in the human body . In medicine, it is crucial for evaluating the safety and efficacy of dapagliflozin by identifying and quantifying impurities . In the pharmaceutical industry, it is used to develop and validate analytical methods for quality control and regulatory compliance .
相似化合物的比较
Dapagliflozin keto impurity can be compared with other impurities and metabolites of dapagliflozin, such as benzylic hydroxy dapagliflozin, oxo dapagliflozin, and desethyl dapagliflozin . These compounds share similar chemical structures but differ in their functional groups and metabolic pathways. For example, benzylic hydroxy dapagliflozin has a hydroxyl group at the benzylic position, while oxo dapagliflozin has a ketone group . Desethyl dapagliflozin lacks an ethyl group compared to the parent compound . These differences can affect their pharmacological properties and potential therapeutic applications.
属性
IUPAC Name |
[2-chloro-5-[(2S,3R,4R,5R,6R)-3,4,5-trihydroxy-6-(hydroxymethyl)oxan-2-yl]phenyl]-(4-ethoxyphenyl)methanone | |
---|---|---|
Source | PubChem | |
URL | https://pubchem.ncbi.nlm.nih.gov | |
Description | Data deposited in or computed by PubChem | |
InChI |
InChI=1S/C21H23ClO7/c1-2-28-13-6-3-11(4-7-13)17(24)14-9-12(5-8-15(14)22)21-20(27)19(26)18(25)16(10-23)29-21/h3-9,16,18-21,23,25-27H,2,10H2,1H3/t16-,18+,19+,20-,21+/m1/s1 | |
Source | PubChem | |
URL | https://pubchem.ncbi.nlm.nih.gov | |
Description | Data deposited in or computed by PubChem | |
InChI Key |
YPBPJGIQJRTYNA-RQSWOZRGSA-N | |
Source | PubChem | |
URL | https://pubchem.ncbi.nlm.nih.gov | |
Description | Data deposited in or computed by PubChem | |
Canonical SMILES |
CCOC1=CC=C(C=C1)C(=O)C2=C(C=CC(=C2)C3C(C(C(C(O3)CO)O)O)O)Cl | |
Source | PubChem | |
URL | https://pubchem.ncbi.nlm.nih.gov | |
Description | Data deposited in or computed by PubChem | |
Isomeric SMILES |
CCOC1=CC=C(C=C1)C(=O)C2=C(C=CC(=C2)[C@H]3[C@@H]([C@H]([C@H]([C@H](O3)CO)O)O)O)Cl | |
Source | PubChem | |
URL | https://pubchem.ncbi.nlm.nih.gov | |
Description | Data deposited in or computed by PubChem | |
Molecular Formula |
C21H23ClO7 | |
Source | PubChem | |
URL | https://pubchem.ncbi.nlm.nih.gov | |
Description | Data deposited in or computed by PubChem | |
Molecular Weight |
422.9 g/mol | |
Source | PubChem | |
URL | https://pubchem.ncbi.nlm.nih.gov | |
Description | Data deposited in or computed by PubChem | |
Retrosynthesis Analysis
AI-Powered Synthesis Planning: Our tool employs the Template_relevance Pistachio, Template_relevance Bkms_metabolic, Template_relevance Pistachio_ringbreaker, Template_relevance Reaxys, Template_relevance Reaxys_biocatalysis model, leveraging a vast database of chemical reactions to predict feasible synthetic routes.
One-Step Synthesis Focus: Specifically designed for one-step synthesis, it provides concise and direct routes for your target compounds, streamlining the synthesis process.
Accurate Predictions: Utilizing the extensive PISTACHIO, BKMS_METABOLIC, PISTACHIO_RINGBREAKER, REAXYS, REAXYS_BIOCATALYSIS database, our tool offers high-accuracy predictions, reflecting the latest in chemical research and data.
Strategy Settings
Precursor scoring | Relevance Heuristic |
---|---|
Min. plausibility | 0.01 |
Model | Template_relevance |
Template Set | Pistachio/Bkms_metabolic/Pistachio_ringbreaker/Reaxys/Reaxys_biocatalysis |
Top-N result to add to graph | 6 |
Feasible Synthetic Routes
体外研究产品的免责声明和信息
请注意,BenchChem 上展示的所有文章和产品信息仅供信息参考。 BenchChem 上可购买的产品专为体外研究设计,这些研究在生物体外进行。体外研究,源自拉丁语 "in glass",涉及在受控实验室环境中使用细胞或组织进行的实验。重要的是要注意,这些产品没有被归类为药物或药品,他们没有得到 FDA 的批准,用于预防、治疗或治愈任何医疗状况、疾病或疾病。我们必须强调,将这些产品以任何形式引入人类或动物的身体都是法律严格禁止的。遵守这些指南对确保研究和实验的法律和道德标准的符合性至关重要。