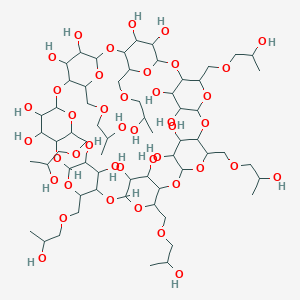
羟丙基-β-环糊精
描述
(2-Hydroxypropyl)-|A-cyclodextrin is a chemically modified derivative of β-cyclodextrin, which is a cyclic oligosaccharide composed of seven glucose units. This compound is known for its ability to enhance the solubility, stability, and bioavailability of various molecules, including drugs. It is widely used in pharmaceutical formulations due to its low toxicity and excellent solubilizing properties .
科学研究应用
(2-Hydroxypropyl)-|A-cyclodextrin has a wide range of scientific research applications:
Pharmaceuticals: It is used to enhance the solubility and bioavailability of poorly soluble drugs, improving their therapeutic efficacy.
Medicine: It has shown potential in treating diseases such as Niemann-Pick type C disease and Alzheimer’s disease by facilitating cholesterol transport and reducing lipid accumulation
作用机制
Target of Action
The primary targets of 2-Hydroxypropyl-β-cyclodextrin (HPβCD) are cholesterol and lipofuscin . HPβCD has shown promise in treating diseases related to cholesterol processing and membrane biology, such as Alzheimer’s disease, Niemann-Pick disease (NPC), age-related macular degeneration, and atherosclerosis . It also targets lipofuscin, an aging biomarker that accumulates within postmitotic cells during aging .
Mode of Action
HPβCD interacts with its targets by binding and liberating cholesterol and oxysterols, shifting the overall cellular cholesterol equilibrium away from the cell . It also transports sequestered cholesterol from the lysosome to the cytosol . In human fibroblasts, HPβCD mitigates the adverse effects of oxidative stress by significantly reducing LDLr and SREBP1 gene expression . In the absence of oxidative stress, HPβCD induces a paradoxical response, increasing cholesterol accumulation via upregulation of cholesterol biosynthesis .
Biochemical Pathways
HPβCD affects the cholesterol-associated pathway . It reduces cholesterol uptake and intracellular accumulation of lipofuscin, an aging biomarker . It also reduces LDLr and SREBP1 gene expression, which are involved in cholesterol metabolism . In the absence of oxidative stress, HPβCD upregulates cholesterol biosynthesis .
Pharmacokinetics
It is known that hpβcd has fast distribution and elimination .
Result of Action
HPβCD reduces age-related lipofuscin accumulation through a cholesterol-associated pathway . It significantly reduces lipofuscin levels in aged LF-loaded, but otherwise healthy, human skin fibroblasts . It also stimulates both proliferation and migration of human umbilical vein endothelial cells (HUVECs) in an endothelial nitric oxide synthase (eNOS)/NO-dependent manner .
Action Environment
The cholesterol content of the treated cell determines which cholesterol pathways, either beneficial or harmful, are responsive to HPβCD . This highlights a previously overlooked therapeutic consideration . The action of HPβCD is also influenced by oxidative stress . In the absence of oxidative stress, HPβCD induces a paradoxical response, increasing cholesterol accumulation .
生化分析
Biochemical Properties
(2-Hydroxypropyl)-β-cyclodextrin plays a crucial role in biochemical reactions by forming inclusion complexes with various hydrophobic molecules. This interaction occurs due to the hydrophobic cavity of the cyclodextrin, which can encapsulate hydrophobic molecules, thereby increasing their solubility in aqueous solutions . The compound interacts with enzymes, proteins, and other biomolecules, such as cholesterol, by forming non-covalent inclusion complexes. These interactions can modulate the activity and stability of the encapsulated molecules, making (2-Hydroxypropyl)-β-cyclodextrin a versatile agent in biochemical research and pharmaceutical formulations .
Cellular Effects
(2-Hydroxypropyl)-β-cyclodextrin has significant effects on various cell types and cellular processes. It is known to influence cell function by modulating cholesterol levels within the cell membrane. This modulation can impact cell signaling pathways, gene expression, and cellular metabolism . For example, in macrophage-derived cell lines, (2-Hydroxypropyl)-β-cyclodextrin has been shown to exert pro-inflammatory effects by altering cholesterol homeostasis and triggering inflammatory signaling pathways . Additionally, it can reduce age-related lipofuscin accumulation in human fibroblasts by affecting cholesterol-associated pathways .
Molecular Mechanism
The molecular mechanism of (2-Hydroxypropyl)-β-cyclodextrin involves its ability to form inclusion complexes with hydrophobic molecules, particularly cholesterol. This binding interaction facilitates the removal of cholesterol from cellular membranes, thereby altering membrane fluidity and function . The compound can also inhibit or activate enzymes involved in cholesterol metabolism, leading to changes in gene expression and cellular responses . These molecular interactions make (2-Hydroxypropyl)-β-cyclodextrin a potent agent for modulating cholesterol-related cellular processes.
Temporal Effects in Laboratory Settings
In laboratory settings, the effects of (2-Hydroxypropyl)-β-cyclodextrin can change over time. The compound is relatively stable, but its long-term effects on cellular function can vary depending on the experimental conditions . For instance, high doses of (2-Hydroxypropyl)-β-cyclodextrin have been associated with rapid onset of ototoxicity in rats, leading to significant destruction of inner and outer hair cells . These temporal effects highlight the importance of carefully monitoring the dosage and duration of exposure in experimental studies.
Dosage Effects in Animal Models
The effects of (2-Hydroxypropyl)-β-cyclodextrin vary with different dosages in animal models. At lower doses, the compound can effectively modulate cholesterol levels and exhibit therapeutic effects without significant toxicity . At higher doses, (2-Hydroxypropyl)-β-cyclodextrin can cause adverse effects, such as ototoxicity and cellular damage . These dosage-dependent effects underscore the need for precise dosing regimens in preclinical and clinical studies to balance efficacy and safety.
Metabolic Pathways
(2-Hydroxypropyl)-β-cyclodextrin is involved in metabolic pathways related to cholesterol metabolism. It interacts with enzymes and cofactors that regulate cholesterol homeostasis, such as low-density lipoprotein receptors and sterol regulatory element-binding proteins . By modulating these pathways, the compound can influence metabolic flux and alter metabolite levels within cells . This makes (2-Hydroxypropyl)-β-cyclodextrin a valuable tool for studying cholesterol metabolism and related disorders.
Transport and Distribution
The transport and distribution of (2-Hydroxypropyl)-β-cyclodextrin within cells and tissues are influenced by its interactions with transporters and binding proteins. The compound can cross the blood-brain barrier and distribute to various regions of the brain and spinal cord when administered intrathecally . Its distribution is also affected by the presence of efflux transporters, which can limit its accumulation in certain tissues . Understanding these transport and distribution mechanisms is crucial for optimizing the therapeutic use of (2-Hydroxypropyl)-β-cyclodextrin.
Subcellular Localization
(2-Hydroxypropyl)-β-cyclodextrin exhibits specific subcellular localization patterns that can affect its activity and function. The compound can localize to lysosomes and other intracellular compartments, where it interacts with cholesterol and other biomolecules . These localization patterns are influenced by targeting signals and post-translational modifications that direct (2-Hydroxypropyl)-β-cyclodextrin to specific cellular compartments . This subcellular localization is essential for understanding the compound’s mechanism of action and optimizing its use in therapeutic applications.
准备方法
Synthetic Routes and Reaction Conditions
(2-Hydroxypropyl)-|A-cyclodextrin is synthesized by the chemical modification of β-cyclodextrin through the reaction with propylene oxide. The reaction typically involves the following steps:
Activation of β-cyclodextrin: β-cyclodextrin is dissolved in an alkaline solution, usually sodium hydroxide, to activate the hydroxyl groups.
Reaction with Propylene Oxide: Propylene oxide is added to the activated β-cyclodextrin solution. .
Industrial Production Methods
Industrial production of (2-Hydroxypropyl)-|A-cyclodextrin follows similar synthetic routes but on a larger scale. The process involves continuous monitoring and optimization of reaction conditions to achieve high yields and purity. The final product is purified through techniques such as crystallization, filtration, and drying .
化学反应分析
Types of Reactions
(2-Hydroxypropyl)-|A-cyclodextrin undergoes various chemical reactions, including:
Inclusion Complex Formation: It forms inclusion complexes with hydrophobic molecules, enhancing their solubility and stability.
Oxidation and Reduction: It can participate in oxidation and reduction reactions, although these are less common.
Substitution Reactions: The hydroxyl groups on the cyclodextrin ring can undergo substitution reactions with various reagents
Common Reagents and Conditions
Oxidizing Agents: Hydrogen peroxide, potassium permanganate.
Reducing Agents: Sodium borohydride, lithium aluminum hydride.
Substitution Reagents: Alkyl halides, acyl chlorides.
Major Products
The major products formed from these reactions include various derivatives of (2-Hydroxypropyl)-|A-cyclodextrin with modified functional groups, which can be tailored for specific applications .
相似化合物的比较
(2-Hydroxypropyl)-|A-cyclodextrin is compared with other cyclodextrin derivatives such as:
Methyl-β-cyclodextrin: Known for its ability to extract cholesterol from cell membranes but has higher toxicity compared to (2-Hydroxypropyl)-|A-cyclodextrin.
Sulfobutyl Ether-β-cyclodextrin: Used for its enhanced solubility and lower toxicity, similar to (2-Hydroxypropyl)-|A-cyclodextrin.
Randomly Methylated-β-cyclodextrin: Offers improved solubility but may have variable properties due to random substitution.
(2-Hydroxypropyl)-|A-cyclodextrin stands out due to its balanced properties of low toxicity, high solubility, and versatility in forming inclusion complexes, making it a preferred choice in various applications .
属性
IUPAC Name |
5,10,15,20,25,30,35-heptakis(2-hydroxypropoxymethyl)-2,4,7,9,12,14,17,19,22,24,27,29,32,34-tetradecaoxaoctacyclo[31.2.2.23,6.28,11.213,16.218,21.223,26.228,31]nonatetracontane-36,37,38,39,40,41,42,43,44,45,46,47,48,49-tetradecol | |
---|---|---|
Details | Computed by Lexichem TK 2.7.0 (PubChem release 2021.05.07) | |
Source | PubChem | |
URL | https://pubchem.ncbi.nlm.nih.gov | |
Description | Data deposited in or computed by PubChem | |
InChI |
InChI=1S/C63H112O42/c1-22(64)8-85-15-29-50-36(71)43(78)57(92-29)100-51-30(16-86-9-23(2)65)94-59(45(80)38(51)73)102-53-32(18-88-11-25(4)67)96-61(47(82)40(53)75)104-55-34(20-90-13-27(6)69)98-63(49(84)42(55)77)105-56-35(21-91-14-28(7)70)97-62(48(83)41(56)76)103-54-33(19-89-12-26(5)68)95-60(46(81)39(54)74)101-52-31(17-87-10-24(3)66)93-58(99-50)44(79)37(52)72/h22-84H,8-21H2,1-7H3 | |
Details | Computed by InChI 1.0.6 (PubChem release 2021.05.07) | |
Source | PubChem | |
URL | https://pubchem.ncbi.nlm.nih.gov | |
Description | Data deposited in or computed by PubChem | |
InChI Key |
ODLHGICHYURWBS-UHFFFAOYSA-N | |
Details | Computed by InChI 1.0.6 (PubChem release 2021.05.07) | |
Source | PubChem | |
URL | https://pubchem.ncbi.nlm.nih.gov | |
Description | Data deposited in or computed by PubChem | |
Canonical SMILES |
CC(COCC1C2C(C(C(O1)OC3C(OC(C(C3O)O)OC4C(OC(C(C4O)O)OC5C(OC(C(C5O)O)OC6C(OC(C(C6O)O)OC7C(OC(C(C7O)O)OC8C(OC(O2)C(C8O)O)COCC(C)O)COCC(C)O)COCC(C)O)COCC(C)O)COCC(C)O)COCC(C)O)O)O)O | |
Details | Computed by OEChem 2.3.0 (PubChem release 2021.05.07) | |
Source | PubChem | |
URL | https://pubchem.ncbi.nlm.nih.gov | |
Description | Data deposited in or computed by PubChem | |
Molecular Formula |
C63H112O42 | |
Details | Computed by PubChem 2.1 (PubChem release 2021.05.07) | |
Source | PubChem | |
URL | https://pubchem.ncbi.nlm.nih.gov | |
Description | Data deposited in or computed by PubChem | |
Molecular Weight |
1541.5 g/mol | |
Details | Computed by PubChem 2.1 (PubChem release 2021.05.07) | |
Source | PubChem | |
URL | https://pubchem.ncbi.nlm.nih.gov | |
Description | Data deposited in or computed by PubChem | |
Physical Description |
Dry Powder; Dry Powder, Liquid; Liquid, White or beige powder; [Sigma-Aldrich MSDS] | |
Record name | .beta.-Cyclodextrin, 2-hydroxypropyl ethers | |
Source | EPA Chemicals under the TSCA | |
URL | https://www.epa.gov/chemicals-under-tsca | |
Description | EPA Chemicals under the Toxic Substances Control Act (TSCA) collection contains information on chemicals and their regulations under TSCA, including non-confidential content from the TSCA Chemical Substance Inventory and Chemical Data Reporting. | |
Record name | 2-Hydroxypropyl-beta-cyclodextrin | |
Source | Haz-Map, Information on Hazardous Chemicals and Occupational Diseases | |
URL | https://haz-map.com/Agents/11476 | |
Description | Haz-Map® is an occupational health database designed for health and safety professionals and for consumers seeking information about the adverse effects of workplace exposures to chemical and biological agents. | |
Explanation | Copyright (c) 2022 Haz-Map(R). All rights reserved. Unless otherwise indicated, all materials from Haz-Map are copyrighted by Haz-Map(R). No part of these materials, either text or image may be used for any purpose other than for personal use. Therefore, reproduction, modification, storage in a retrieval system or retransmission, in any form or by any means, electronic, mechanical or otherwise, for reasons other than personal use, is strictly prohibited without prior written permission. | |
CAS No. |
128446-35-5 | |
Record name | .beta.-Cyclodextrin, 2-hydroxypropyl ethers | |
Source | EPA Chemicals under the TSCA | |
URL | https://www.epa.gov/chemicals-under-tsca | |
Description | EPA Chemicals under the Toxic Substances Control Act (TSCA) collection contains information on chemicals and their regulations under TSCA, including non-confidential content from the TSCA Chemical Substance Inventory and Chemical Data Reporting. | |
Record name | β-Cyclodextrin, 2-hydroxypropyl ethers | |
Source | European Chemicals Agency (ECHA) | |
URL | https://echa.europa.eu/substance-information/-/substanceinfo/100.119.815 | |
Description | The European Chemicals Agency (ECHA) is an agency of the European Union which is the driving force among regulatory authorities in implementing the EU's groundbreaking chemicals legislation for the benefit of human health and the environment as well as for innovation and competitiveness. | |
Explanation | Use of the information, documents and data from the ECHA website is subject to the terms and conditions of this Legal Notice, and subject to other binding limitations provided for under applicable law, the information, documents and data made available on the ECHA website may be reproduced, distributed and/or used, totally or in part, for non-commercial purposes provided that ECHA is acknowledged as the source: "Source: European Chemicals Agency, http://echa.europa.eu/". Such acknowledgement must be included in each copy of the material. ECHA permits and encourages organisations and individuals to create links to the ECHA website under the following cumulative conditions: Links can only be made to webpages that provide a link to the Legal Notice page. | |
Retrosynthesis Analysis
AI-Powered Synthesis Planning: Our tool employs the Template_relevance Pistachio, Template_relevance Bkms_metabolic, Template_relevance Pistachio_ringbreaker, Template_relevance Reaxys, Template_relevance Reaxys_biocatalysis model, leveraging a vast database of chemical reactions to predict feasible synthetic routes.
One-Step Synthesis Focus: Specifically designed for one-step synthesis, it provides concise and direct routes for your target compounds, streamlining the synthesis process.
Accurate Predictions: Utilizing the extensive PISTACHIO, BKMS_METABOLIC, PISTACHIO_RINGBREAKER, REAXYS, REAXYS_BIOCATALYSIS database, our tool offers high-accuracy predictions, reflecting the latest in chemical research and data.
Strategy Settings
Precursor scoring | Relevance Heuristic |
---|---|
Min. plausibility | 0.01 |
Model | Template_relevance |
Template Set | Pistachio/Bkms_metabolic/Pistachio_ringbreaker/Reaxys/Reaxys_biocatalysis |
Top-N result to add to graph | 6 |
Feasible Synthetic Routes
体外研究产品的免责声明和信息
请注意,BenchChem 上展示的所有文章和产品信息仅供信息参考。 BenchChem 上可购买的产品专为体外研究设计,这些研究在生物体外进行。体外研究,源自拉丁语 "in glass",涉及在受控实验室环境中使用细胞或组织进行的实验。重要的是要注意,这些产品没有被归类为药物或药品,他们没有得到 FDA 的批准,用于预防、治疗或治愈任何医疗状况、疾病或疾病。我们必须强调,将这些产品以任何形式引入人类或动物的身体都是法律严格禁止的。遵守这些指南对确保研究和实验的法律和道德标准的符合性至关重要。