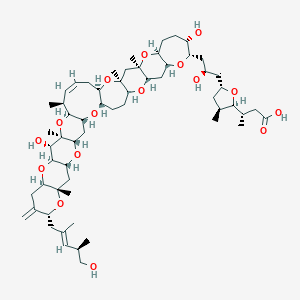
Gambieric acid A
- 点击 快速询问 获取最新报价。
- 提供有竞争力价格的高质量产品,您可以更专注于研究。
描述
Gambieric acid A is a natural product found in Gambierdiscus toxicus with data available.
科学研究应用
Antifungal Activity
Gambieric acid A exhibits remarkable antifungal properties , particularly against Aspergillus niger. Studies indicate that its antifungal potency is approximately 2000 times greater than that of amphotericin B , a widely used antifungal agent . This high efficacy positions this compound as a promising candidate for developing new antifungal therapies, especially in an era of increasing resistance to conventional antifungal drugs.
Structure-Activity Relationship Studies
Research on this compound has led to extensive investigations into its structure-activity relationships (SAR). The total synthesis of this compound has facilitated the creation of analogs that help elucidate the molecular features responsible for its biological activity. For instance, studies have demonstrated that modifications in the molecular structure can significantly influence its antifungal and antiproliferative activities .
Table 1: Structure-Activity Relationships of this compound
Modification | Effect on Activity | Reference |
---|---|---|
C9 and C11 stereogenic centers | Altered potency against Aspergillus niger | |
Ring modifications | Impact on cytotoxicity in cancer cell lines |
Potential Therapeutic Applications
Beyond its antifungal properties, this compound is being explored for broader therapeutic applications. Its unique chemical structure and biological activity suggest potential roles in treating various conditions:
- Cancer : Preliminary studies indicate that this compound may exhibit cytotoxic effects against certain cancer cell lines, warranting further exploration into its mechanisms and efficacy as an anticancer agent .
- Growth Regulation : There is evidence suggesting that this compound could act as an endogenous growth-regulating factor for Gambierdiscus toxicus, indicating a role in ecological interactions and possibly therapeutic applications related to growth modulation .
Synthetic Studies and Challenges
The complexity of this compound's structure has posed significant challenges for synthetic chemists. Recent advancements have led to successful total syntheses, which not only provide access to this compound but also enable the exploration of its derivatives . These synthetic approaches often involve sophisticated techniques such as Suzuki-Miyaura coupling and ring-closing metathesis, highlighting the intricate nature of producing this compound in the laboratory.
Case Studies
Several case studies illustrate the applications and research surrounding this compound:
- Total Synthesis and Biological Evaluation : In a landmark study, researchers achieved the first total synthesis of this compound, followed by comprehensive biological evaluations that confirmed its potent antifungal activity. This work laid the groundwork for future SAR studies and potential therapeutic applications .
- Antifungal Efficacy Against Drug-Resistant Strains : Investigations into the efficacy of this compound against drug-resistant fungal strains have shown promising results, suggesting it could be developed into a novel treatment option for resistant infections .
属性
CAS 编号 |
138434-64-7 |
---|---|
分子式 |
C59H92O16 |
分子量 |
1057.3 g/mol |
IUPAC 名称 |
(3S)-3-[(2R,3S,5R)-5-[(2R)-3-[(1S,3R,5S,7R,9S,11R,14R,16S,17S,18R,20S,21S,22Z,25R,27S,29R,31S,34S,35S,37R,39S,41R)-17,34-dihydroxy-11-[(E,4R)-5-hydroxy-2,4-dimethylpent-2-enyl]-9,18,21,27,29-pentamethyl-12-methylidene-2,6,10,15,19,26,30,36,40-nonaoxanonacyclo[23.18.0.03,20.05,18.07,16.09,14.027,41.029,39.031,37]tritetracont-22-en-35-yl]-2-hydroxypropyl]-3-methyloxolan-2-yl]butanoic acid |
InChI |
InChI=1S/C59H92O16/c1-30(18-31(2)28-60)19-42-33(4)21-48-56(7,74-42)27-46-54(71-48)55(65)59(10)50(69-46)26-45-53(75-59)32(3)12-11-13-40-39(67-45)16-17-47-57(8,72-40)29-58(9)49(70-47)25-44-41(73-58)15-14-38(62)43(68-44)24-36(61)23-37-20-34(5)52(66-37)35(6)22-51(63)64/h11-12,18,31-32,34-50,52-55,60-62,65H,4,13-17,19-29H2,1-3,5-10H3,(H,63,64)/b12-11-,30-18+/t31-,32+,34+,35+,36-,37-,38+,39+,40-,41+,42-,43+,44-,45-,46-,47-,48-,49+,50+,52-,53+,54-,55+,56+,57+,58-,59+/m1/s1 |
InChI 键 |
MSZMCMVREIGRAG-PXDMALBISA-N |
SMILES |
CC1CC(OC1C(C)CC(=O)O)CC(CC2C(CCC3C(O2)CC4C(O3)(CC5(C(O4)CCC6C(O5)CC=CC(C7C(O6)CC8C(O7)(C(C9C(O8)CC1(C(O9)CC(=C)C(O1)CC(=CC(C)CO)C)C)O)C)C)C)C)O)O |
手性 SMILES |
C[C@H]1C[C@@H](O[C@H]1[C@@H](C)CC(=O)O)C[C@H](C[C@H]2[C@H](CC[C@H]3[C@H](O2)C[C@H]4[C@](O3)(C[C@]5([C@H](O4)CC[C@H]6[C@H](O5)C/C=C\[C@@H]([C@H]7[C@H](O6)C[C@H]8[C@](O7)([C@H]([C@H]9[C@H](O8)C[C@]1([C@H](O9)CC(=C)[C@H](O1)C/C(=C/[C@@H](C)CO)/C)C)O)C)C)C)C)O)O |
规范 SMILES |
CC1CC(OC1C(C)CC(=O)O)CC(CC2C(CCC3C(O2)CC4C(O3)(CC5(C(O4)CCC6C(O5)CC=CC(C7C(O6)CC8C(O7)(C(C9C(O8)CC1(C(O9)CC(=C)C(O1)CC(=CC(C)CO)C)C)O)C)C)C)C)O)O |
同义词 |
Gambieric acid A |
产品来源 |
United States |
Retrosynthesis Analysis
AI-Powered Synthesis Planning: Our tool employs the Template_relevance Pistachio, Template_relevance Bkms_metabolic, Template_relevance Pistachio_ringbreaker, Template_relevance Reaxys, Template_relevance Reaxys_biocatalysis model, leveraging a vast database of chemical reactions to predict feasible synthetic routes.
One-Step Synthesis Focus: Specifically designed for one-step synthesis, it provides concise and direct routes for your target compounds, streamlining the synthesis process.
Accurate Predictions: Utilizing the extensive PISTACHIO, BKMS_METABOLIC, PISTACHIO_RINGBREAKER, REAXYS, REAXYS_BIOCATALYSIS database, our tool offers high-accuracy predictions, reflecting the latest in chemical research and data.
Strategy Settings
Precursor scoring | Relevance Heuristic |
---|---|
Min. plausibility | 0.01 |
Model | Template_relevance |
Template Set | Pistachio/Bkms_metabolic/Pistachio_ringbreaker/Reaxys/Reaxys_biocatalysis |
Top-N result to add to graph | 6 |
Feasible Synthetic Routes
体外研究产品的免责声明和信息
请注意,BenchChem 上展示的所有文章和产品信息仅供信息参考。 BenchChem 上可购买的产品专为体外研究设计,这些研究在生物体外进行。体外研究,源自拉丁语 "in glass",涉及在受控实验室环境中使用细胞或组织进行的实验。重要的是要注意,这些产品没有被归类为药物或药品,他们没有得到 FDA 的批准,用于预防、治疗或治愈任何医疗状况、疾病或疾病。我们必须强调,将这些产品以任何形式引入人类或动物的身体都是法律严格禁止的。遵守这些指南对确保研究和实验的法律和道德标准的符合性至关重要。