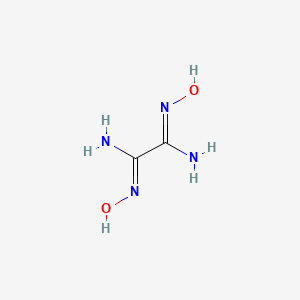
Diaminoglyoxime
描述
Diaminoglyoxime is a versatile organic compound known for its applications in various fields of chemistry, including synthesis, catalysis, and material science. It is characterized by the presence of two amino groups attached to a glyoxime moiety, making it a valuable reagent in organic synthesis.
Synthetic Routes and Reaction Conditions:
One-Pot Synthesis: this compound can be synthesized through a one-pot reaction involving glyoxal and hydroxylamine hydrochloride in the presence of aqueous sodium hydroxide. This method is cost-effective and yields a high product.
Industrial Production: On an industrial scale, the synthesis involves optimizing reaction conditions such as temperature, solvent choice, and stoichiometry to achieve higher yields and purity.
Types of Reactions:
Oxidation: this compound can undergo oxidation reactions to form various oxidized products.
Reduction: Reduction reactions can convert this compound into its reduced forms.
Substitution: It can participate in substitution reactions with various reagents.
Common Reagents and Conditions:
Oxidation: Common oxidizing agents include hydrogen peroxide and potassium permanganate.
Reduction: Reducing agents like sodium borohydride are often used.
Substitution: Various ketones and aldehydes can be used as reactants.
Major Products Formed:
Oxidation Products: Various oxo-compounds.
Reduction Products: Reduced forms of this compound.
Substitution Products: Bis(1,2,4-oxadiazoles), 1,2,4-oxadiazolyl-quinazolines, and 1,2,4-oxadiazolyl-benzothiazinones.
作用机制
Target of Action
Diaminoglyoxime (DAG) is primarily used in the synthesis of various compounds, including bis(1,2,4-oxadiazoles), 1,2,4-oxadiazolyl-quinazolines, and 1,2,4-oxadiazolyl-benzothiazinones . These compounds have a broad spectrum of biological activities, including anti-inflammatory, antimicrobial, antioxidant, anticancer, and antihypertensive activities .
Mode of Action
DAG interacts with its targets through a variety of mechanisms. For instance, in the synthesis of bis(1,2,4-oxadiazoles), 1,2,4-oxadiazolyl-quinazolines, and 1,2,4-oxadiazolyl-benzothiazinones, DAG reacts with various ketones and methyl 2-aminobenzoate, 2-amino-5-chlorophenyl)(phenyl)methanone, and 2-mercapto benzoic acid in acetic acid . The reaction progresses via nucleophilic attack of the mercapto group on the oxime carbon and of the amine group on the carboxylic acid .
Biochemical Pathways
It has been found to be a useful substructure for creating new high-density and energetic materials composed exclusively of hydrogen, carbon, oxygen, and nitrogen atoms .
Pharmacokinetics
It’s known that dag can be converted into nh3 and co2, either through direct hydrolysis or through pyrolysis and polymerization, and then further hydrolysis .
Result of Action
The result of DAG’s action depends on the specific context. For instance, in a Ni–DAG–g-C3N4 complex, DAG contributes to a 21-fold enhancement of photocatalytic activity under irradiation, leading to efficient H2 evolution through photocatalytic ethanol splitting .
Action Environment
The action of DAG can be influenced by environmental factors. For example, DAG was reacted in water at 150–400°C and at 27.5 MPa pressure in a metal Ti tubing reactor, leading to its conversion into NH3 and CO2 . This suggests that high temperature and pressure can significantly influence the action and stability of DAG.
生化分析
Biochemical Properties
Diaminoglyoxime plays a significant role in biochemical reactions, particularly in the synthesis of biologically active compounds. It interacts with various enzymes, proteins, and other biomolecules. For instance, this compound has been used in the synthesis of bis(1,2,4-oxadiazoles), which exhibit a range of biological activities such as anti-inflammatory, antidiabetic, and antimicrobial properties . The interactions between this compound and these biomolecules are primarily based on its ability to form stable complexes and facilitate specific chemical transformations.
Cellular Effects
This compound influences various cellular processes and functions. It has been observed to enhance photocatalytic activity in certain cellular environments. For example, a novel Ni–this compound–g-C3N4 complex has shown a 21-fold enhancement in photocatalytic activity for hydrogen evolution through ethanol splitting . This indicates that this compound can significantly impact cell signaling pathways, gene expression, and cellular metabolism by facilitating efficient energy conversion processes.
Molecular Mechanism
At the molecular level, this compound exerts its effects through specific binding interactions with biomolecules. It has been reported to form stable complexes with transition metals such as nickel, which enhances its catalytic properties . These interactions can lead to enzyme activation or inhibition, depending on the context. Additionally, this compound’s ability to form stable complexes with various biomolecules can result in changes in gene expression and other molecular processes.
Temporal Effects in Laboratory Settings
In laboratory settings, the effects of this compound can change over time. Studies have shown that this compound can influence the thermal behaviors and decomposition kinetics of propellants . Its stability and degradation over time are crucial factors in determining its long-term effects on cellular function. For instance, this compound has been found to alter the thermal decomposition mechanism and kinetic parameters of propellants, indicating its potential as a ballistic modifier .
Dosage Effects in Animal Models
The effects of this compound vary with different dosages in animal models. While specific studies on this compound’s dosage effects are limited, general principles from similar compounds suggest that varying dosages can lead to different threshold effects and potential toxicities. High doses of this compound may result in adverse effects, while lower doses could be beneficial for specific biochemical applications .
Metabolic Pathways
This compound is involved in various metabolic pathways, particularly those related to energy conversion and catalysis. It interacts with enzymes and cofactors that facilitate these processes. For example, the Ni–this compound–g-C3N4 complex enhances photocatalytic activity through a ligand-to-metal charge transfer mechanism . This interaction highlights the role of this compound in modulating metabolic flux and influencing metabolite levels.
Transport and Distribution
Within cells and tissues, this compound is transported and distributed through specific transporters and binding proteins. Its ability to form stable complexes with transition metals suggests that it may be localized in areas with high metal ion concentrations . This localization can affect its accumulation and overall activity within the cellular environment.
Subcellular Localization
This compound’s subcellular localization is influenced by its chemical properties and interactions with other biomolecules. It may be directed to specific compartments or organelles through targeting signals or post-translational modifications. For instance, its interaction with transition metals can lead to its localization in areas where these metals are abundant, thereby influencing its activity and function .
科学研究应用
Diaminoglyoxime is widely used in scientific research due to its versatility:
Chemistry: It serves as a reagent in the synthesis of bis(1,2,4-oxadiazoles), 1,2,4-oxadiazolyl-quinazolines, and 1,2,4-oxadiazolyl-benzothiazinones.
Biology: It is used in the study of enzyme mechanisms and as a ligand in metal complexes.
Medicine: this compound derivatives are explored for their potential therapeutic properties.
Industry: It is used as a catalyst in various industrial processes, including hydrogen generation from formic acid decomposition.
相似化合物的比较
Glyoxal
Hydroxylamine
Oxadiazoles
Does this cover everything you were looking for, or is there something specific you'd like to dive deeper into?
属性
IUPAC Name |
1-N',2-N'-dihydroxyethanediimidamide | |
---|---|---|
Details | Computed by Lexichem TK 2.7.0 (PubChem release 2021.05.07) | |
Source | PubChem | |
URL | https://pubchem.ncbi.nlm.nih.gov | |
Description | Data deposited in or computed by PubChem | |
InChI |
InChI=1S/C2H6N4O2/c3-1(5-7)2(4)6-8/h7-8H,(H2,3,5)(H2,4,6) | |
Details | Computed by InChI 1.0.6 (PubChem release 2021.05.07) | |
Source | PubChem | |
URL | https://pubchem.ncbi.nlm.nih.gov | |
Description | Data deposited in or computed by PubChem | |
InChI Key |
BFXWFQSYMVKOCJ-UHFFFAOYSA-N | |
Details | Computed by InChI 1.0.6 (PubChem release 2021.05.07) | |
Source | PubChem | |
URL | https://pubchem.ncbi.nlm.nih.gov | |
Description | Data deposited in or computed by PubChem | |
Canonical SMILES |
C(=NO)(C(=NO)N)N | |
Details | Computed by OEChem 2.3.0 (PubChem release 2021.05.07) | |
Source | PubChem | |
URL | https://pubchem.ncbi.nlm.nih.gov | |
Description | Data deposited in or computed by PubChem | |
Molecular Formula |
C2H6N4O2 | |
Details | Computed by PubChem 2.1 (PubChem release 2021.05.07) | |
Source | PubChem | |
URL | https://pubchem.ncbi.nlm.nih.gov | |
Description | Data deposited in or computed by PubChem | |
DSSTOX Substance ID |
DTXSID20878687 | |
Record name | Ethanediimidamide, N,N''-dihydroxy- | |
Source | EPA DSSTox | |
URL | https://comptox.epa.gov/dashboard/DTXSID20878687 | |
Description | DSSTox provides a high quality public chemistry resource for supporting improved predictive toxicology. | |
Molecular Weight |
118.10 g/mol | |
Details | Computed by PubChem 2.1 (PubChem release 2021.05.07) | |
Source | PubChem | |
URL | https://pubchem.ncbi.nlm.nih.gov | |
Description | Data deposited in or computed by PubChem | |
CAS No. |
2580-79-2 | |
Record name | Ethanediimidamide, N,N''-dihydroxy- | |
Source | EPA DSSTox | |
URL | https://comptox.epa.gov/dashboard/DTXSID20878687 | |
Description | DSSTox provides a high quality public chemistry resource for supporting improved predictive toxicology. | |
Retrosynthesis Analysis
AI-Powered Synthesis Planning: Our tool employs the Template_relevance Pistachio, Template_relevance Bkms_metabolic, Template_relevance Pistachio_ringbreaker, Template_relevance Reaxys, Template_relevance Reaxys_biocatalysis model, leveraging a vast database of chemical reactions to predict feasible synthetic routes.
One-Step Synthesis Focus: Specifically designed for one-step synthesis, it provides concise and direct routes for your target compounds, streamlining the synthesis process.
Accurate Predictions: Utilizing the extensive PISTACHIO, BKMS_METABOLIC, PISTACHIO_RINGBREAKER, REAXYS, REAXYS_BIOCATALYSIS database, our tool offers high-accuracy predictions, reflecting the latest in chemical research and data.
Strategy Settings
Precursor scoring | Relevance Heuristic |
---|---|
Min. plausibility | 0.01 |
Model | Template_relevance |
Template Set | Pistachio/Bkms_metabolic/Pistachio_ringbreaker/Reaxys/Reaxys_biocatalysis |
Top-N result to add to graph | 6 |
Feasible Synthetic Routes
Q1: What is the molecular formula and weight of Diaminoglyoxime?
A1: this compound has the molecular formula C2H6N4O2 and a molecular weight of 118.09 g/mol. []
Q2: What spectroscopic techniques are typically used to characterize this compound and its derivatives?
A2: Common spectroscopic techniques employed include:
- Infrared (IR) Spectroscopy: Provides information about the functional groups present in the molecule, particularly the characteristic stretching vibrations of O-H, C=N, and N-H bonds. [, , , , , , , ]
- Nuclear Magnetic Resonance (NMR) Spectroscopy: Offers insights into the structure and connectivity of atoms within the molecule, with 1H NMR and 13C NMR being frequently utilized. [, , , , , ]
- UV-Vis Spectroscopy: Helps determine the electronic transitions within the molecule and study the formation and properties of metal complexes. [, , ]
- Mass Spectrometry (MS): Used to determine the molecular weight and fragmentation patterns of the compound, aiding in structural elucidation. [, , , ]
Q3: What is the typical conformation of this compound in its free form?
A3: this compound usually exists in the trans-anti form, as confirmed by IR and 1H NMR data. []
Q4: Why is this compound considered a versatile ligand in coordination chemistry?
A4: DAG acts as a vicinal dioxime ligand, meaning it possesses two oxime groups (C=N-OH) in close proximity. These oxime groups can chelate metal ions, forming stable five-membered rings. [, , , ]
Q5: What types of metal complexes are commonly formed with this compound?
A5: DAG forms complexes with a variety of transition metals, including but not limited to:
- Square-Planar Complexes: Frequently observed with Ni(II), Cu(II), and Pd(II) ions. [, , , , , , ]
- Octahedral Complexes: Reported with Co(II) ions. [, ]
- Polymeric Complexes: Observed with Cu(II), Co(II), and Ni(II) ions when bridging ligands or specific reaction conditions are employed. [, ]
- Heteropentanuclear Complexes: Formed when combining Ni(II) complexes of DAG with Cu(II) salts and monodentate ligands. []
Q6: How does the structure of this compound influence the geometry of its metal complexes?
A6: The presence of two oxime groups in a trans configuration facilitates the formation of square-planar complexes by allowing the oxime nitrogen atoms to occupy adjacent coordination sites around the metal center. [, , , , , , ]
Q7: Can the amino groups of this compound participate in coordination?
A7: While the primary coordination sites are the oxime nitrogen atoms, studies suggest that amino groups can participate in coordination, especially at higher pH values, leading to the formation of polynuclear species. [, ]
Q8: What are some notable catalytic applications of this compound and its derivatives?
A8: DAG and its derivatives have shown promise in various catalytic applications, such as:
- Suzuki-Miyaura Cross-Coupling Reactions: Palladium complexes of DAG, like this compound palladium (II) chloride (PdCl2/DAG complex), efficiently catalyze the formation of biaryl compounds from aryl halides and phenylboronic acids, even under aerobic and phosphine-free conditions in aqueous solutions. []
- Photocatalytic Ethanol Splitting: Ni-diaminoglyoxime-g-C3N4 (Ni-DAG-CN) complexes exhibit enhanced photocatalytic activity for hydrogen evolution from ethanol splitting, attributed to a ligand-to-metal charge transfer (LMCT) mechanism. []
- Formic Acid Dehydrogenation: Iridium catalysts bearing dioxime-derived ligands, inspired by DAG's structure, demonstrate exceptional efficiency and stability in dehydrogenating formic acid for hydrogen generation. []
Q9: How do the structural features of this compound contribute to its catalytic properties?
A9: * Chelating Ability: The chelating ability of the vicinal dioxime moiety allows DAG to form stable complexes with catalytically active transition metals like palladium, nickel, and iridium. * Electronic Properties: The electronic structure of the DAG ligand can be modified through substitutions on the amine nitrogen atoms, influencing the electron density at the metal center and consequently, the catalytic activity. []* Stability: The robust nature of the DAG ligand, especially when coordinated to a metal, contributes to the stability and reusability of the catalysts. [, , ]
Q10: What are the common synthetic routes to this compound?
A10: DAG is typically synthesized by reacting glyoxal with hydroxylamine hydrochloride in the presence of a base such as sodium hydroxide. This reaction proceeds through the formation of glyoxime as an intermediate, which further reacts with hydroxylamine to yield DAG. [, , , ]
Q11: What are the advantages of microwave-assisted synthesis of this compound and its derivatives?
A11: Microwave irradiation significantly reduces the reaction times required for DAG synthesis, leading to a faster and more efficient process. [, ]
Q12: What are some challenges in the synthesis of this compound?
A12: The synthesis of DAG can be prone to thermal runaway reactions, resulting in lower yields and safety concerns. Additionally, purification steps, such as recrystallization, are often required to obtain high-purity DAG. [, ]
Q13: What are some applications of this compound in materials science?
A13:
- High Energy Density Materials (HEDMs): DAG serves as a key precursor for synthesizing 3,4-diaminofurazan (DAF), a vital building block for high-energy density materials. [, , ]
- Melt-Castable Explosives: Derivatives of DAG have been explored for the development of melt-castable explosives, owing to their suitable thermal properties and insensitivity to external stimuli. []
- Liquid Crystalline Materials: Certain metal complexes of DAG derivatives exhibit liquid crystalline properties, making them potentially useful in display technologies and other applications requiring anisotropic materials. []
- Molecular Conductors: DAG complexes with d8 transition metals have shown potential as molecular conductors, particularly when subjected to partial oxidation. []
Q14: What are the potential advantages of using this compound derivatives in the development of high energy density materials?
A14: DAG derivatives, particularly those containing furazan rings, offer several advantages in HEDM development, including:
- High Nitrogen Content: Leads to enhanced energy release upon decomposition. [, ]
- Good Thermal Stability: Ensures safe handling and storage of the materials. [, ]
- Tunable Properties: Structural modifications of DAG derivatives allow for the fine-tuning of properties such as density, oxygen balance, and sensitivity. []
Q15: How can the properties of this compound metal complexes be further modified for specific applications?
A15: The properties of DAG metal complexes can be tailored by:
- Varying the Metal Center: Different metal ions impart distinct electronic and magnetic properties to the complexes. [, ]
- Introducing Substituents: Modifications to the amine groups of DAG can influence solubility, electronic properties, and steric hindrance around the metal center. [, ]
- Using Bridging Ligands: Incorporating bridging ligands can lead to the formation of polynuclear complexes with unique properties. [, ]
Q16: How is computational chemistry employed in the study of this compound and its derivatives?
A16: Computational methods play a crucial role in understanding and predicting the properties of DAG and its derivatives. * Density Functional Theory (DFT): Used to optimize molecular geometries, calculate electronic structures, and investigate reaction mechanisms. [, ]* Molecular Dynamics (MD) Simulations: Employed to study the dynamic behavior of DAG complexes in solution and solid-state, providing insights into their stability and interactions with other molecules.* Quantitative Structure-Activity Relationship (QSAR) Studies: Used to correlate the structural features of DAG derivatives with their biological or physicochemical properties, aiding in the design of new compounds with improved properties.
体外研究产品的免责声明和信息
请注意,BenchChem 上展示的所有文章和产品信息仅供信息参考。 BenchChem 上可购买的产品专为体外研究设计,这些研究在生物体外进行。体外研究,源自拉丁语 "in glass",涉及在受控实验室环境中使用细胞或组织进行的实验。重要的是要注意,这些产品没有被归类为药物或药品,他们没有得到 FDA 的批准,用于预防、治疗或治愈任何医疗状况、疾病或疾病。我们必须强调,将这些产品以任何形式引入人类或动物的身体都是法律严格禁止的。遵守这些指南对确保研究和实验的法律和道德标准的符合性至关重要。