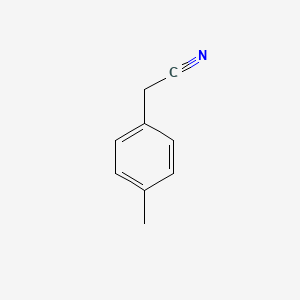
4-Methylbenzyl cyanide
描述
4-Methylbenzyl cyanide: 4-Methylphenylacetonitrile or p-Tolylacetonitrile , is an organic compound with the molecular formula C9H9N . It is a clear, colorless to faintly yellow liquid with a boiling point of 242-243°C and a density of 0.992 g/mL at 25°C . This compound is used as an intermediate in the synthesis of various pharmaceuticals, fragrances, and other organic compounds .
准备方法
Synthetic Routes and Reaction Conditions: 4-Methylbenzyl cyanide can be synthesized through several methods:
Kolbe Nitrile Synthesis: This method involves the reaction of benzyl chloride with sodium cyanide to produce benzyl cyanide, which can then be methylated to yield this compound.
Industrial Production Methods: Industrial production of this compound typically involves large-scale implementation of the above synthetic routes, with optimizations for yield and purity. The use of continuous flow reactors and advanced purification techniques ensures the efficient production of high-purity this compound .
化学反应分析
Types of Reactions: 4-Methylbenzyl cyanide undergoes various chemical reactions, including:
Reduction: Reduction of this compound can yield 4-Methylbenzylamine using reducing agents like lithium aluminum hydride.
Substitution: The nitrile group can be hydrolyzed to form 4-Methylphenylacetic acid under acidic or basic conditions.
Common Reagents and Conditions:
Oxidation: Potassium permanganate (KMnO4) in acidic conditions.
Reduction: Lithium aluminum hydride (LiAlH4) in anhydrous ether.
Hydrolysis: Acidic or basic aqueous solutions.
Major Products:
Oxidation: 4-Methylbenzoic acid.
Reduction: 4-Methylbenzylamine.
Hydrolysis: 4-Methylphenylacetic acid.
科学研究应用
4-Methylbenzyl cyanide has several applications in scientific research:
作用机制
The mechanism of action of 4-Methylbenzyl cyanide involves its reactivity as a nitrile compound. The nitrile group can undergo various transformations, such as hydrolysis, reduction, and substitution, leading to the formation of different products. These reactions often involve nucleophilic attack on the carbon atom of the nitrile group, followed by subsequent steps depending on the reaction conditions .
相似化合物的比较
Benzyl cyanide: Similar structure but lacks the methyl group on the benzene ring.
4-Methoxybenzyl cyanide: Contains a methoxy group instead of a methyl group.
4-Nitrophenylacetonitrile: Contains a nitro group instead of a methyl group.
Uniqueness of 4-Methylbenzyl cyanide: this compound is unique due to the presence of the methyl group on the benzene ring, which influences its reactivity and the types of reactions it undergoes. This methyl group can affect the electron density on the benzene ring, making it more reactive towards certain electrophilic and nucleophilic reagents .
属性
IUPAC Name |
2-(4-methylphenyl)acetonitrile | |
---|---|---|
Source | PubChem | |
URL | https://pubchem.ncbi.nlm.nih.gov | |
Description | Data deposited in or computed by PubChem | |
InChI |
InChI=1S/C9H9N/c1-8-2-4-9(5-3-8)6-7-10/h2-5H,6H2,1H3 | |
Source | PubChem | |
URL | https://pubchem.ncbi.nlm.nih.gov | |
Description | Data deposited in or computed by PubChem | |
InChI Key |
RNHKXHKUKJXLAU-UHFFFAOYSA-N | |
Source | PubChem | |
URL | https://pubchem.ncbi.nlm.nih.gov | |
Description | Data deposited in or computed by PubChem | |
Canonical SMILES |
CC1=CC=C(C=C1)CC#N | |
Source | PubChem | |
URL | https://pubchem.ncbi.nlm.nih.gov | |
Description | Data deposited in or computed by PubChem | |
Molecular Formula |
C9H9N | |
Source | PubChem | |
URL | https://pubchem.ncbi.nlm.nih.gov | |
Description | Data deposited in or computed by PubChem | |
DSSTOX Substance ID |
DTXSID90183674 | |
Record name | p-Tolylacetonitrile | |
Source | EPA DSSTox | |
URL | https://comptox.epa.gov/dashboard/DTXSID90183674 | |
Description | DSSTox provides a high quality public chemistry resource for supporting improved predictive toxicology. | |
Molecular Weight |
131.17 g/mol | |
Source | PubChem | |
URL | https://pubchem.ncbi.nlm.nih.gov | |
Description | Data deposited in or computed by PubChem | |
CAS No. |
2947-61-7 | |
Record name | 4-Methylphenylacetonitrile | |
Source | CAS Common Chemistry | |
URL | https://commonchemistry.cas.org/detail?cas_rn=2947-61-7 | |
Description | CAS Common Chemistry is an open community resource for accessing chemical information. Nearly 500,000 chemical substances from CAS REGISTRY cover areas of community interest, including common and frequently regulated chemicals, and those relevant to high school and undergraduate chemistry classes. This chemical information, curated by our expert scientists, is provided in alignment with our mission as a division of the American Chemical Society. | |
Explanation | The data from CAS Common Chemistry is provided under a CC-BY-NC 4.0 license, unless otherwise stated. | |
Record name | p-Tolylacetonitrile | |
Source | ChemIDplus | |
URL | https://pubchem.ncbi.nlm.nih.gov/substance/?source=chemidplus&sourceid=0002947617 | |
Description | ChemIDplus is a free, web search system that provides access to the structure and nomenclature authority files used for the identification of chemical substances cited in National Library of Medicine (NLM) databases, including the TOXNET system. | |
Record name | p-Tolylacetonitrile | |
Source | EPA DSSTox | |
URL | https://comptox.epa.gov/dashboard/DTXSID90183674 | |
Description | DSSTox provides a high quality public chemistry resource for supporting improved predictive toxicology. | |
Record name | p-tolylacetonitrile | |
Source | European Chemicals Agency (ECHA) | |
URL | https://echa.europa.eu/substance-information/-/substanceinfo/100.019.058 | |
Description | The European Chemicals Agency (ECHA) is an agency of the European Union which is the driving force among regulatory authorities in implementing the EU's groundbreaking chemicals legislation for the benefit of human health and the environment as well as for innovation and competitiveness. | |
Explanation | Use of the information, documents and data from the ECHA website is subject to the terms and conditions of this Legal Notice, and subject to other binding limitations provided for under applicable law, the information, documents and data made available on the ECHA website may be reproduced, distributed and/or used, totally or in part, for non-commercial purposes provided that ECHA is acknowledged as the source: "Source: European Chemicals Agency, http://echa.europa.eu/". Such acknowledgement must be included in each copy of the material. ECHA permits and encourages organisations and individuals to create links to the ECHA website under the following cumulative conditions: Links can only be made to webpages that provide a link to the Legal Notice page. | |
Record name | P-TOLYLACETONITRILE | |
Source | FDA Global Substance Registration System (GSRS) | |
URL | https://gsrs.ncats.nih.gov/ginas/app/beta/substances/5LWN3C33SM | |
Description | The FDA Global Substance Registration System (GSRS) enables the efficient and accurate exchange of information on what substances are in regulated products. Instead of relying on names, which vary across regulatory domains, countries, and regions, the GSRS knowledge base makes it possible for substances to be defined by standardized, scientific descriptions. | |
Explanation | Unless otherwise noted, the contents of the FDA website (www.fda.gov), both text and graphics, are not copyrighted. They are in the public domain and may be republished, reprinted and otherwise used freely by anyone without the need to obtain permission from FDA. Credit to the U.S. Food and Drug Administration as the source is appreciated but not required. | |
Retrosynthesis Analysis
AI-Powered Synthesis Planning: Our tool employs the Template_relevance Pistachio, Template_relevance Bkms_metabolic, Template_relevance Pistachio_ringbreaker, Template_relevance Reaxys, Template_relevance Reaxys_biocatalysis model, leveraging a vast database of chemical reactions to predict feasible synthetic routes.
One-Step Synthesis Focus: Specifically designed for one-step synthesis, it provides concise and direct routes for your target compounds, streamlining the synthesis process.
Accurate Predictions: Utilizing the extensive PISTACHIO, BKMS_METABOLIC, PISTACHIO_RINGBREAKER, REAXYS, REAXYS_BIOCATALYSIS database, our tool offers high-accuracy predictions, reflecting the latest in chemical research and data.
Strategy Settings
Precursor scoring | Relevance Heuristic |
---|---|
Min. plausibility | 0.01 |
Model | Template_relevance |
Template Set | Pistachio/Bkms_metabolic/Pistachio_ringbreaker/Reaxys/Reaxys_biocatalysis |
Top-N result to add to graph | 6 |
Feasible Synthetic Routes
Q1: How does 4-Methylbenzyl cyanide react with potassium superoxide in the presence of 18-crown-6?
A: According to research [], this compound reacts with potassium superoxide (KO2) in the presence of 18-crown-6 under ultrasonic conditions to yield α-(4-methylbenzoyl)-4-methylbenzyl cyanide and 4-methylbenzoic acid. Interestingly, the reaction outcome differs significantly from benzyl cyanide, which produces α-benzoylbenzyl cyanide under the same conditions. This difference highlights the impact of the methyl substitution on the aromatic ring. When the reaction with this compound is carried out with simple stirring instead of sonication, no products are formed. This suggests that ultrasound plays a crucial role in activating the reaction. While reflux conditions can also facilitate the reaction, they lead to lower yields compared to sonication.
Q2: Can this compound be used to synthesize fluorescent compounds?
A: While the provided research [, ] doesn't directly address this question, it does offer insight into the reactivity of this compound. The paper focuses on nucleophilic substitution reactions of 5-nitrobenzimidazole derivatives with this compound under basic conditions []. This reaction leads to the formation of 3-alkyl-8-methyl-3H-imidazo[4,5-a]acridines, a class of fluorescent heterocyclic compounds. While the paper doesn't explicitly state that this compound contributes to the fluorescence of the final product, its incorporation into the molecular structure could influence the compound's photophysical properties. Further research would be needed to determine the specific role of this compound in the fluorescence of these newly synthesized compounds.
体外研究产品的免责声明和信息
请注意,BenchChem 上展示的所有文章和产品信息仅供信息参考。 BenchChem 上可购买的产品专为体外研究设计,这些研究在生物体外进行。体外研究,源自拉丁语 "in glass",涉及在受控实验室环境中使用细胞或组织进行的实验。重要的是要注意,这些产品没有被归类为药物或药品,他们没有得到 FDA 的批准,用于预防、治疗或治愈任何医疗状况、疾病或疾病。我们必须强调,将这些产品以任何形式引入人类或动物的身体都是法律严格禁止的。遵守这些指南对确保研究和实验的法律和道德标准的符合性至关重要。