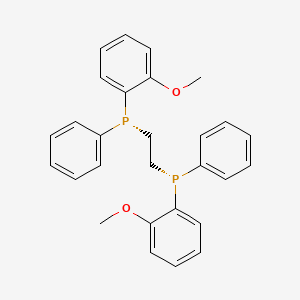
(R,R)-Dipamp
描述
(R,R)-Dipamp, also known as (R,R)-1,2-bis[(2-methoxyphenyl)phenylphosphino]ethane, is a chiral diphosphine ligand widely used in asymmetric synthesis. It is particularly known for its application in catalytic asymmetric hydrogenation reactions. The compound’s chiral nature allows it to induce enantioselectivity in various chemical transformations, making it a valuable tool in the synthesis of enantiomerically pure compounds.
准备方法
Synthetic Routes and Reaction Conditions: The synthesis of (R,R)-Dipamp typically involves the reaction of (R,R)-1,2-diaminoethane with chlorodiphenylphosphine in the presence of a base. The reaction proceeds through the formation of an intermediate phosphine, which is then further reacted with 2-methoxyphenyl lithium to yield the final product. The reaction conditions often require an inert atmosphere, such as nitrogen or argon, to prevent oxidation of the phosphine groups.
Industrial Production Methods: Industrial production of this compound follows similar synthetic routes but on a larger scale. The process involves careful control of reaction parameters, such as temperature, pressure, and reactant concentrations, to ensure high yield and purity of the product. The use of automated reactors and continuous flow systems can enhance the efficiency and scalability of the production process.
化学反应分析
Types of Reactions: (R,R)-Dipamp undergoes various types of chemical reactions, including:
Oxidation: The phosphine groups in this compound can be oxidized to form phosphine oxides.
Reduction: The compound can participate in reduction reactions, particularly in catalytic hydrogenation processes.
Substitution: The ligand can undergo substitution reactions where the phosphine groups are replaced by other functional groups.
Common Reagents and Conditions:
Oxidation: Common oxidizing agents include hydrogen peroxide and oxygen.
Reduction: Hydrogen gas is often used in the presence of a metal catalyst, such as palladium or rhodium.
Substitution: Reagents like alkyl halides or acyl chlorides can be used for substitution reactions.
Major Products:
Oxidation: Phosphine oxides.
Reduction: Reduced organic substrates, such as alkenes to alkanes.
Substitution: Substituted phosphine derivatives.
科学研究应用
(R,R)-Dipamp has a wide range of applications in scientific research, including:
Chemistry: It is extensively used as a chiral ligand in asymmetric catalysis, particularly in hydrogenation reactions to produce enantiomerically pure compounds.
Biology: The compound’s ability to induce chirality makes it useful in the synthesis of biologically active molecules, such as pharmaceuticals and agrochemicals.
Medicine: this compound is employed in the synthesis of chiral drugs, where enantioselectivity is crucial for the drug’s efficacy and safety.
Industry: The compound is used in the production of fine chemicals and specialty materials, where precise control over stereochemistry is required.
作用机制
The mechanism by which (R,R)-Dipamp exerts its effects involves its coordination to a metal center, forming a chiral metal-ligand complex. This complex can then interact with substrates in a stereoselective manner, facilitating asymmetric transformations. The molecular targets include various metal catalysts, such as rhodium, palladium, and platinum, which mediate the catalytic processes. The pathways involved typically include the formation of metal-substrate intermediates, followed by enantioselective reactions that yield chiral products.
相似化合物的比较
(S,S)-Dipamp: The enantiomer of (R,R)-Dipamp, used in similar applications but induces opposite chirality.
BINAP: Another chiral diphosphine ligand widely used in asymmetric catalysis.
DIOP: A chiral diphosphine ligand with a different backbone structure but similar applications in asymmetric synthesis.
Uniqueness: this compound is unique due to its high enantioselectivity and versatility in various catalytic processes. Its ability to form stable complexes with a wide range of metal catalysts makes it a preferred choice in many asymmetric synthesis applications. The compound’s specific chiral configuration allows for precise control over the stereochemistry of the products, which is essential in the synthesis of enantiomerically pure compounds.
属性
IUPAC Name |
(R)-(2-methoxyphenyl)-[2-[(2-methoxyphenyl)-phenylphosphanyl]ethyl]-phenylphosphane | |
---|---|---|
Source | PubChem | |
URL | https://pubchem.ncbi.nlm.nih.gov | |
Description | Data deposited in or computed by PubChem | |
InChI |
InChI=1S/C28H28O2P2/c1-29-25-17-9-11-19-27(25)31(23-13-5-3-6-14-23)21-22-32(24-15-7-4-8-16-24)28-20-12-10-18-26(28)30-2/h3-20H,21-22H2,1-2H3/t31-,32-/m1/s1 | |
Source | PubChem | |
URL | https://pubchem.ncbi.nlm.nih.gov | |
Description | Data deposited in or computed by PubChem | |
InChI Key |
QKZWXPLBVCKXNQ-ROJLCIKYSA-N | |
Source | PubChem | |
URL | https://pubchem.ncbi.nlm.nih.gov | |
Description | Data deposited in or computed by PubChem | |
Canonical SMILES |
COC1=CC=CC=C1P(CCP(C2=CC=CC=C2)C3=CC=CC=C3OC)C4=CC=CC=C4 | |
Source | PubChem | |
URL | https://pubchem.ncbi.nlm.nih.gov | |
Description | Data deposited in or computed by PubChem | |
Isomeric SMILES |
COC1=CC=CC=C1[P@](CC[P@](C2=CC=CC=C2)C3=CC=CC=C3OC)C4=CC=CC=C4 | |
Source | PubChem | |
URL | https://pubchem.ncbi.nlm.nih.gov | |
Description | Data deposited in or computed by PubChem | |
Molecular Formula |
C28H28O2P2 | |
Source | PubChem | |
URL | https://pubchem.ncbi.nlm.nih.gov | |
Description | Data deposited in or computed by PubChem | |
DSSTOX Substance ID |
DTXSID401027540 | |
Record name | (R,R)-Dipamp | |
Source | EPA DSSTox | |
URL | https://comptox.epa.gov/dashboard/DTXSID401027540 | |
Description | DSSTox provides a high quality public chemistry resource for supporting improved predictive toxicology. | |
Molecular Weight |
458.5 g/mol | |
Source | PubChem | |
URL | https://pubchem.ncbi.nlm.nih.gov | |
Description | Data deposited in or computed by PubChem | |
CAS No. |
55739-58-7 | |
Record name | Dipamp, (R,R)- | |
Source | ChemIDplus | |
URL | https://pubchem.ncbi.nlm.nih.gov/substance/?source=chemidplus&sourceid=0055739587 | |
Description | ChemIDplus is a free, web search system that provides access to the structure and nomenclature authority files used for the identification of chemical substances cited in National Library of Medicine (NLM) databases, including the TOXNET system. | |
Record name | (R,R)-Dipamp | |
Source | EPA DSSTox | |
URL | https://comptox.epa.gov/dashboard/DTXSID401027540 | |
Description | DSSTox provides a high quality public chemistry resource for supporting improved predictive toxicology. | |
Record name | (R,R)-1,2-Bis[(2-methoxyphenyl)phenylphosphino]ethane | |
Source | European Chemicals Agency (ECHA) | |
URL | https://echa.europa.eu/information-on-chemicals | |
Description | The European Chemicals Agency (ECHA) is an agency of the European Union which is the driving force among regulatory authorities in implementing the EU's groundbreaking chemicals legislation for the benefit of human health and the environment as well as for innovation and competitiveness. | |
Explanation | Use of the information, documents and data from the ECHA website is subject to the terms and conditions of this Legal Notice, and subject to other binding limitations provided for under applicable law, the information, documents and data made available on the ECHA website may be reproduced, distributed and/or used, totally or in part, for non-commercial purposes provided that ECHA is acknowledged as the source: "Source: European Chemicals Agency, http://echa.europa.eu/". Such acknowledgement must be included in each copy of the material. ECHA permits and encourages organisations and individuals to create links to the ECHA website under the following cumulative conditions: Links can only be made to webpages that provide a link to the Legal Notice page. | |
Record name | DIPAMP, (R,R)- | |
Source | FDA Global Substance Registration System (GSRS) | |
URL | https://gsrs.ncats.nih.gov/ginas/app/beta/substances/BLJ831OWLW | |
Description | The FDA Global Substance Registration System (GSRS) enables the efficient and accurate exchange of information on what substances are in regulated products. Instead of relying on names, which vary across regulatory domains, countries, and regions, the GSRS knowledge base makes it possible for substances to be defined by standardized, scientific descriptions. | |
Explanation | Unless otherwise noted, the contents of the FDA website (www.fda.gov), both text and graphics, are not copyrighted. They are in the public domain and may be republished, reprinted and otherwise used freely by anyone without the need to obtain permission from FDA. Credit to the U.S. Food and Drug Administration as the source is appreciated but not required. | |
Retrosynthesis Analysis
AI-Powered Synthesis Planning: Our tool employs the Template_relevance Pistachio, Template_relevance Bkms_metabolic, Template_relevance Pistachio_ringbreaker, Template_relevance Reaxys, Template_relevance Reaxys_biocatalysis model, leveraging a vast database of chemical reactions to predict feasible synthetic routes.
One-Step Synthesis Focus: Specifically designed for one-step synthesis, it provides concise and direct routes for your target compounds, streamlining the synthesis process.
Accurate Predictions: Utilizing the extensive PISTACHIO, BKMS_METABOLIC, PISTACHIO_RINGBREAKER, REAXYS, REAXYS_BIOCATALYSIS database, our tool offers high-accuracy predictions, reflecting the latest in chemical research and data.
Strategy Settings
Precursor scoring | Relevance Heuristic |
---|---|
Min. plausibility | 0.01 |
Model | Template_relevance |
Template Set | Pistachio/Bkms_metabolic/Pistachio_ringbreaker/Reaxys/Reaxys_biocatalysis |
Top-N result to add to graph | 6 |
Feasible Synthetic Routes
体外研究产品的免责声明和信息
请注意,BenchChem 上展示的所有文章和产品信息仅供信息参考。 BenchChem 上可购买的产品专为体外研究设计,这些研究在生物体外进行。体外研究,源自拉丁语 "in glass",涉及在受控实验室环境中使用细胞或组织进行的实验。重要的是要注意,这些产品没有被归类为药物或药品,他们没有得到 FDA 的批准,用于预防、治疗或治愈任何医疗状况、疾病或疾病。我们必须强调,将这些产品以任何形式引入人类或动物的身体都是法律严格禁止的。遵守这些指南对确保研究和实验的法律和道德标准的符合性至关重要。