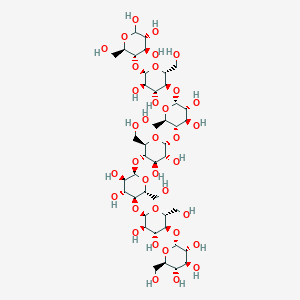
Maltoheptaose
描述
Maltoheptaose (G7) is a linear oligosaccharide composed of seven α-1,4-linked D-glucose units, forming a non-cyclic structure distinct from cyclodextrins (CDs). It serves as a critical model substrate in enzymology, structural biology, and analytical chemistry due to its intermediate chain length and solubility. Key applications include:
- Enzyme Kinetics: Used to study glycosyltransferases, amylases, and disproportionating enzymes (D-enzymes) due to its role in starch metabolism .
- Structural Studies: Co-crystallized with proteins like SusF (Bacteroides thetaiotaomicron) to elucidate carbohydrate-binding mechanisms .
- Analytical Chemistry: Employed as an internal standard in mass spectrometry (MS) for N-linked glycan quantification, leveraging its hydrophilic interaction liquid chromatography (HILIC) compatibility despite lacking N-acetylglucosamine or sialic acid residues .
- Material Science: Integrated into block copolymers (e.g., this compound-b-PCL) for self-assembling micelles, demonstrating its utility in nanotechnology .
准备方法
Synthetic Routes and Reaction Conditions: Maltoheptaose can be synthesized through enzymatic processes. One common method involves the use of cyclodextrin glucotransferase and cyclomaltodextrinase in a one-pot cascade reaction. The optimal conditions for this reaction are a pH of 7.0 and a temperature of 30°C, with the presence of calcium ions. The sequential addition of these enzymes results in a higher conversion rate compared to simultaneous addition .
Another method involves the use of cyclodextrinase derived from Thermococcus species, expressed in Bacillus subtilis. This enzyme exhibits high substrate specificity for cyclodextrins and can achieve a high yield of this compound under optimal conditions of 90°C and pH 5.5 .
Industrial Production Methods: In industrial settings, this compound is typically produced from starch using enzymatic hydrolysis. The process involves the use of specific enzymes that break down starch into maltooligosaccharides, including this compound. The enzymes used in this process include cyclodextrin glucotransferase and cyclomaltodextrinase, which facilitate the conversion of starch into this compound .
化学反应分析
Types of Reactions: Maltoheptaose undergoes various chemical reactions, including hydrolysis, oxidation, and glycosylation.
Common Reagents and Conditions:
Hydrolysis: Enzymatic hydrolysis using amylases can break down this compound into smaller glucose units.
Oxidation: Oxidizing agents such as sodium periodate can oxidize the hydroxyl groups on the glucose units.
Glycosylation: Glycosylation reactions can be carried out using glycosyltransferases to attach additional sugar units to this compound.
Major Products:
Hydrolysis: Produces smaller glucose units such as maltose and glucose.
Oxidation: Produces oxidized derivatives of this compound with altered functional groups.
Glycosylation: Produces extended oligosaccharides with additional sugar units
科学研究应用
Drug Delivery Systems
Maltoheptaose has been utilized as a key component in the development of advanced drug delivery systems, particularly in nanocarrier formulations.
Nanoparticles for Cancer Therapy:
Recent studies have demonstrated the potential of this compound-b-polystyrene (MH-b-PS) nanoparticles as effective carriers for lipophilic anticancer drugs like tamoxifen. These nanoparticles exhibit high encapsulation efficiency (80.9%) and enhanced cytotoxicity against MCF-7 breast cancer cells compared to free tamoxifen. The mechanism involves the interaction of this compound with glucose transporters on cancer cells, facilitating internalization and improving therapeutic outcomes .
Glycoliposomes for Antibiotic Delivery:
this compound-presenting glycoliposomes have been synthesized to enhance the delivery of antibiotics such as rifampicin. These glycoliposomes selectively target bacterial cells while minimizing interactions with mammalian cells, demonstrating significant potential for treating bacterial infections .
Nutritional Applications
In the food industry, this compound is recognized for its functional properties and health benefits.
Prebiotic Effects:
As a prebiotic, this compound can promote the growth of beneficial gut bacteria, contributing to improved digestive health. Its ability to resist digestion in the upper gastrointestinal tract allows it to reach the colon intact, where it serves as a substrate for fermentation by gut microbiota .
Nutritional Supplements:
this compound is often included in nutritional products due to its digestibility and energy-providing capabilities. It is particularly useful in formulations aimed at athletes or individuals requiring quick energy sources .
Research and Analytical Applications
This compound serves as an important tool in carbohydrate chemistry and analytical techniques.
Carbohydrate Analysis:
In research settings, this compound is employed as a standard in mass spectrometry and capillary electrophoresis for the analysis of carbohydrates and glyconjugates. Its distinct mass-to-charge ratio facilitates sensitive detection methods, enhancing the accuracy of carbohydrate profiling .
Case Study 1: this compound Nanoparticles in Cancer Treatment
Researchers designed MH-b-PS nanoparticles loaded with tamoxifen to evaluate their effectiveness against breast cancer cells. The study found that these nanoparticles not only improved drug delivery efficiency but also exhibited a biphasic drug release profile, indicating potential for sustained therapeutic effects .
Case Study 2: Targeted Antibiotic Delivery with Glycoliposomes
In another study, this compound-functionalized glycoliposomes were shown to effectively deliver rifampicin to Mycobacterium species while sparing mammalian cells. This targeted approach resulted in significantly enhanced antibacterial activity compared to conventional delivery methods .
作用机制
Maltoheptaose exerts its effects primarily through its interaction with enzymes involved in carbohydrate metabolism. It serves as a substrate for various amylases and glycosyltransferases, facilitating the breakdown and synthesis of complex carbohydrates. The molecular targets include enzymes such as cyclodextrin glucotransferase and cyclomaltodextrinase, which catalyze the conversion of this compound into other oligosaccharides .
相似化合物的比较
Cyclodextrins (CDs)
Structural Differences :
- Maltoheptaose is linear, whereas β-CD (cyclothis compound) is cyclic, with α-1,4-glucose units forming a rigid toroidal structure.
- Permethylation of β-CD enhances hydrophobic cavity interactions, while permethylated this compound exhibits altered gas-phase fragmentation pathways in MS .
Functional Differences :
- Binding Under Osmotic Stress : Both β-CD and this compound exclude water molecules during ligand binding, following the Hofmeister series. However, β-CD’s cyclic structure results in greater salt-dependent water exclusion (e.g., 15–20 water molecules excluded for β-CD vs. 12–16 for this compound) .
- Mass Spectrometry : β-CD forms host-guest complexes with nucleobases, while this compound undergoes fragmentation pathways influenced by its linearity, producing distinct MS/MS patterns .
Table 1: Comparison of this compound and β-Cyclodextrin
Shorter Maltooligosaccharides (G3–G6)
Enzyme Kinetics :
- D-Enzyme Affinity : The Km for maltotriose (G3) is 5.5 mM, while this compound (G7) has a lower Km (2.6 mM), indicating higher substrate affinity for longer chains .
- Temperature Optima : Maximal activity for D-enzyme occurs at 40°C with G3 vs. 45°C with G7, reflecting chain-length-dependent thermal adaptation .
Transport Through Maltoporin (LamB) :
- This compound serves as a reference for sugar flux rates. Longer maltooligosaccharides (e.g., G8–G9) exhibit similar rate constants (k-1) but differ in binding saturation (40 mM for high-affinity sugars) .
Table 2: Kinetic Parameters of Maltooligosaccharides
Substrate | Km (mM) | Temperature Optimum (°C) | Relative Flux (LamB) | Reference |
---|---|---|---|---|
Maltotriose | 5.5 | 40 | 0.3 | |
This compound | 2.6 | 45 | 1.0 (reference) |
Branched and Modified Oligosaccharides
- Maltotetraosyl-α-1,6-Maltoheptaose : TreX hydrolyzes this branched substrate into this compound and maltotetraose, unlike linear this compound, which resists branching-specific cleavage .
- 4,6-α-Glucanotransferase (GTFB) Products: Incubation with this compound yields linear oligomers with α-1,6 linkages (18–33% in products), contrasting with glycogen’s α-1,4/α-1,6 structure .
N-Linked Glycans
While this compound is used as an internal standard for N-glycan quantification, it lacks N-acetylglucosamine and sialic acid, leading to partial mismatches in hydrophilicity and ionization efficiency. This limits its accuracy in MS-based relative quantification compared to isotopically labeled glycans .
生物活性
Maltoheptaose, a maltooligosaccharide composed of seven glucose units linked by α-(1,4) glycosidic bonds, has garnered attention for its biological activities, particularly in immunomodulation and antimicrobial effects. This article explores the various biological activities associated with this compound, supported by research findings, data tables, and case studies.
Immunomodulatory Effects
Research indicates that this compound plays a significant role in modulating immune responses. A pivotal study demonstrated that this compound binds to Toll-like receptor 2 (TLR-2) on monocytes, leading to enhanced immune activation. This binding significantly increases the expression of immune markers such as CD69, CD80, and CD86, as well as cytokines like IL-12 and TNF-α in human peripheral blood mononuclear cells (hPBMCs) .
The mechanism through which this compound exerts its immunomodulatory effects involves several steps:
- Binding to TLR-2 : this compound interacts with TLR-2 on immune cells, triggering signaling pathways that enhance immune activation.
- Cytokine Production : Following activation, there is a notable increase in the production of Th1 cytokines (IL-12 and TNF-α), which are crucial for the immune response.
- Cellular Activation : The study found that this compound directly activates monocytes, leading to increased expression of surface markers associated with immune activation.
Antimicrobial Activity
In addition to its immunomodulatory properties, this compound has been investigated for its antimicrobial potential. A study involving rifampicin-loaded glycoliposomes showed that the incorporation of this compound significantly enhanced the antimicrobial activity against Escherichia coli. The results indicated up to a 2-log reduction in bacterial colony-forming units at 4 times the minimum inhibitory concentration (MIC) after 24 hours .
Data Tables
The following table summarizes key findings related to the biological activity of this compound:
Case Studies
Several case studies have highlighted the practical applications and implications of this compound in health and disease management:
- Wheatgrass Supplementation : A study explored the effects of wheatgrass-derived oligosaccharides, including this compound, on immune function. Participants who consumed wheatgrass showed increased levels of immune markers and improved overall health outcomes .
- Drug Delivery Systems : Research into nanoscale glycoliposomes incorporating this compound demonstrated improved drug delivery efficiency and antimicrobial action against resistant bacterial strains. This finding suggests potential applications in developing more effective antibiotic therapies .
常见问题
Basic Research Questions
Q. What are the optimal chromatographic methods for purifying maltoheptaose, and how do retention times vary with solvent systems?
- Methodological Answer : Size-exclusion chromatography (SEC) and high-performance anion-exchange chromatography (HPAEC) are widely used. SEC separates this compound based on hydrodynamic radius, while HPAEC leverages its anionic properties under alkaline conditions. Retention times depend on solvent composition (e.g., acetonitrile/water gradients in SEC or NaOH/NaOAc gradients in HPAEC). Calibration with oligosaccharide standards is critical for accurate identification .
Q. How can MALDI-TOF mass spectrometry be standardized to characterize this compound structural integrity?
- Methodological Answer : Matrix selection (e.g., 2,5-dihydroxybenzoic acid) and cationization agents (e.g., sodium or potassium adducts) must be optimized to reduce fragmentation. Internal calibration with known oligosaccharides ensures mass accuracy. Post-source decay (PSD) analysis can further confirm glycosidic linkage patterns by comparing fragmentation profiles to synthetic standards .
Q. What spectroscopic techniques are most reliable for distinguishing this compound from other linear α-1,4-glucans?
- Methodological Answer : Nuclear magnetic resonance (NMR) spectroscopy, particularly -NMR, resolves anomeric carbon signals (δ 100–102 ppm for α-1,4 linkages). Comparing chemical shifts to databases (e.g., CASPER) or synthetic analogs validates purity. Infrared (IR) spectroscopy can also differentiate this compound via O-H stretching (3200–3600 cm) and glycosidic bond vibrations (900–1200 cm) .
Advanced Research Questions
Q. How do collision-induced dissociation (CID) parameters affect this compound fragmentation patterns in gas-phase studies?
- Methodological Answer : Low-energy CID (10–50 eV) primarily cleaves glycosidic bonds, yielding Y- and B-type ions, while higher-energy CID (>50 eV) promotes cross-ring cleavages (e.g., , ions). Calibrating collision energy and gas pressure (e.g., using argon vs. helium) is essential to control fragmentation reproducibility. Comparative studies with isotopically labeled analogs can resolve ambiguities in fragment assignment .
Q. What methodological challenges arise when quantifying this compound’s immunomodulatory effects via Toll-like receptor (TLR) signaling pathways?
- Methodological Answer : Contamination with endotoxins (e.g., LPS) must be ruled out using limulus amebocyte lysate (LAL) assays. Dose-response curves (e.g., 0.1–10 μM) in THP-1 monocytes, coupled with TLR-2 knockout controls, validate specificity. mRNA quantification (e.g., qRT-PCR for IL-6/TNF-α) should normalize to housekeeping genes (e.g., GAPDH) and include kinetic studies to account for transient signaling .
Q. How can molecular dynamics (MD) simulations be applied to predict this compound’s binding energetics with cyclodextrins or lectins?
- Methodological Answer : Force fields (e.g., GLYCAM06) parameterize carbohydrate interactions. Simulations should run for ≥100 ns with explicit solvent models (e.g., TIP3P water) to stabilize hydrogen-bond networks. Binding free energies (ΔG) calculated via MM-PBSA or umbrella sampling must be validated against experimental data (e.g., isothermal titration calorimetry) to resolve discrepancies between predicted and observed affinities .
Q. Data Contradiction and Reproducibility
Q. How should researchers address discrepancies in reported this compound solubility across aqueous and organic solvents?
- Methodological Answer : Solubility studies must standardize temperature (e.g., 25°C), agitation methods, and solvent purity. Conflicting data often arise from undefined oligomer polydispersity or residual salts. Repeating experiments with this compound certified for monodispersity (e.g., via SEC-MALS) and reporting detailed solvent preparation protocols (e.g., degassing, filtration) enhances reproducibility .
Q. What strategies mitigate variability in this compound’s enzymatic hydrolysis rates across studies?
- Methodological Answer : Enzyme-to-substrate ratios (e.g., 1:10–1:100 w/w for α-amylase) and buffer conditions (pH, Ca concentration) must be rigorously controlled. Parallel assays with maltopentaose or maltohexaose as controls differentiate substrate specificity. Real-time monitoring (e.g., HPLC or reducing-sugar assays) reduces endpoint variability .
Q. Suggested Analytical Parameters Table
属性
IUPAC Name |
2-[6-[6-[6-[6-[4,5-dihydroxy-2-(hydroxymethyl)-6-[4,5,6-trihydroxy-2-(hydroxymethyl)oxan-3-yl]oxyoxan-3-yl]oxy-4,5-dihydroxy-2-(hydroxymethyl)oxan-3-yl]oxy-4,5-dihydroxy-2-(hydroxymethyl)oxan-3-yl]oxy-4,5-dihydroxy-2-(hydroxymethyl)oxan-3-yl]oxy-4,5-dihydroxy-2-(hydroxymethyl)oxan-3-yl]oxy-6-(hydroxymethyl)oxane-3,4,5-triol | |
---|---|---|
Details | Computed by LexiChem 2.6.6 (PubChem release 2019.06.18) | |
Source | PubChem | |
URL | https://pubchem.ncbi.nlm.nih.gov | |
Description | Data deposited in or computed by PubChem | |
InChI |
InChI=1S/C42H72O36/c43-1-8-15(50)16(51)24(59)37(67-8)74-31-10(3-45)69-39(26(61)18(31)53)76-33-12(5-47)71-41(28(63)20(33)55)78-35-14(7-49)72-42(29(64)22(35)57)77-34-13(6-48)70-40(27(62)21(34)56)75-32-11(4-46)68-38(25(60)19(32)54)73-30-9(2-44)66-36(65)23(58)17(30)52/h8-65H,1-7H2 | |
Details | Computed by InChI 1.0.5 (PubChem release 2019.06.18) | |
Source | PubChem | |
URL | https://pubchem.ncbi.nlm.nih.gov | |
Description | Data deposited in or computed by PubChem | |
InChI Key |
BNABBHGYYMZMOA-UHFFFAOYSA-N | |
Details | Computed by InChI 1.0.5 (PubChem release 2019.06.18) | |
Source | PubChem | |
URL | https://pubchem.ncbi.nlm.nih.gov | |
Description | Data deposited in or computed by PubChem | |
Canonical SMILES |
C(C1C(C(C(C(O1)OC2C(OC(C(C2O)O)OC3C(OC(C(C3O)O)OC4C(OC(C(C4O)O)OC5C(OC(C(C5O)O)OC6C(OC(C(C6O)O)OC7C(OC(C(C7O)O)O)CO)CO)CO)CO)CO)CO)O)O)O)O | |
Details | Computed by OEChem 2.1.5 (PubChem release 2019.06.18) | |
Source | PubChem | |
URL | https://pubchem.ncbi.nlm.nih.gov | |
Description | Data deposited in or computed by PubChem | |
Molecular Formula |
C42H72O36 | |
Details | Computed by PubChem 2.1 (PubChem release 2019.06.18) | |
Source | PubChem | |
URL | https://pubchem.ncbi.nlm.nih.gov | |
Description | Data deposited in or computed by PubChem | |
DSSTOX Substance ID |
DTXSID80956117 | |
Record name | Hexopyranosyl-(1->4)hexopyranosyl-(1->4)hexopyranosyl-(1->4)hexopyranosyl-(1->4)hexopyranosyl-(1->4)hexopyranosyl-(1->4)hexopyranose | |
Source | EPA DSSTox | |
URL | https://comptox.epa.gov/dashboard/DTXSID80956117 | |
Description | DSSTox provides a high quality public chemistry resource for supporting improved predictive toxicology. | |
Molecular Weight |
1153.0 g/mol | |
Details | Computed by PubChem 2.1 (PubChem release 2021.05.07) | |
Source | PubChem | |
URL | https://pubchem.ncbi.nlm.nih.gov | |
Description | Data deposited in or computed by PubChem | |
CAS No. |
34620-78-5 | |
Record name | Hexopyranosyl-(1->4)hexopyranosyl-(1->4)hexopyranosyl-(1->4)hexopyranosyl-(1->4)hexopyranosyl-(1->4)hexopyranosyl-(1->4)hexopyranose | |
Source | EPA DSSTox | |
URL | https://comptox.epa.gov/dashboard/DTXSID80956117 | |
Description | DSSTox provides a high quality public chemistry resource for supporting improved predictive toxicology. | |
Record name | D-Glucose, O-α-d-glucopyranosyl-(1→4)-O-α-d-glucopyranosyl-(1→4)-O-α-d-glucopyranosyl-(1→4)-O-α-d-glucopyranosyl-(1→4)-O-α-d-glucopyranosyl-(1→4)-O-α-d-glucopyranosyl-(1→4)- | |
Source | European Chemicals Agency (ECHA) | |
URL | https://echa.europa.eu/substance-information/-/substanceinfo/100.047.367 | |
Description | The European Chemicals Agency (ECHA) is an agency of the European Union which is the driving force among regulatory authorities in implementing the EU's groundbreaking chemicals legislation for the benefit of human health and the environment as well as for innovation and competitiveness. | |
Explanation | Use of the information, documents and data from the ECHA website is subject to the terms and conditions of this Legal Notice, and subject to other binding limitations provided for under applicable law, the information, documents and data made available on the ECHA website may be reproduced, distributed and/or used, totally or in part, for non-commercial purposes provided that ECHA is acknowledged as the source: "Source: European Chemicals Agency, http://echa.europa.eu/". Such acknowledgement must be included in each copy of the material. ECHA permits and encourages organisations and individuals to create links to the ECHA website under the following cumulative conditions: Links can only be made to webpages that provide a link to the Legal Notice page. | |
体外研究产品的免责声明和信息
请注意,BenchChem 上展示的所有文章和产品信息仅供信息参考。 BenchChem 上可购买的产品专为体外研究设计,这些研究在生物体外进行。体外研究,源自拉丁语 "in glass",涉及在受控实验室环境中使用细胞或组织进行的实验。重要的是要注意,这些产品没有被归类为药物或药品,他们没有得到 FDA 的批准,用于预防、治疗或治愈任何医疗状况、疾病或疾病。我们必须强调,将这些产品以任何形式引入人类或动物的身体都是法律严格禁止的。遵守这些指南对确保研究和实验的法律和道德标准的符合性至关重要。