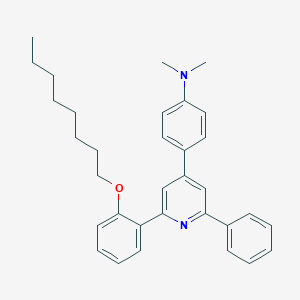
N,N-Dimethyl-4-(2-(2-(octyloxy)phenyl)-6-phenylpyridin-4-yl)aniline
货号:
B130935
CAS 编号:
144190-25-0
分子量:
478.7 g/mol
InChI 键:
GOCLJAKNIYYTMF-UHFFFAOYSA-N
注意:
仅供研究使用。不适用于人类或兽医用途。
现货
- 点击 快速询问 获取最新报价。
- 提供有竞争力价格的高质量产品,您可以更专注于研究。
描述
“N,N-Dimethyl-4-(2-(2-(octyloxy)phenyl)-6-phenylpyridin-4-yl)aniline” is a complex organic compound. It likely contains a pyridine ring (a six-membered ring with five carbon atoms and one nitrogen atom), an aniline group (a phenyl group attached to an amino group), and an octyloxy group (an eight-carbon chain attached to an oxygen atom) based on its name .
Molecular Structure Analysis
The molecular structure of this compound would likely be quite complex, given the presence of multiple aromatic rings and the long octyloxy chain. The exact structure would depend on the positions of these groups on the pyridine ring .Chemical Reactions Analysis
As an organic compound containing several functional groups, “this compound” would likely undergo a variety of chemical reactions. These could include electrophilic aromatic substitution reactions on the phenyl and pyridine rings, as well as reactions involving the amino group .作用机制
未来方向
属性
IUPAC Name |
N,N-dimethyl-4-[2-(2-octoxyphenyl)-6-phenylpyridin-4-yl]aniline |
Source
|
---|---|---|
Source | PubChem | |
URL | https://pubchem.ncbi.nlm.nih.gov | |
Description | Data deposited in or computed by PubChem | |
InChI |
InChI=1S/C33H38N2O/c1-4-5-6-7-8-14-23-36-33-18-13-12-17-30(33)32-25-28(26-19-21-29(22-20-26)35(2)3)24-31(34-32)27-15-10-9-11-16-27/h9-13,15-22,24-25H,4-8,14,23H2,1-3H3 |
Source
|
Source | PubChem | |
URL | https://pubchem.ncbi.nlm.nih.gov | |
Description | Data deposited in or computed by PubChem | |
InChI Key |
GOCLJAKNIYYTMF-UHFFFAOYSA-N |
Source
|
Source | PubChem | |
URL | https://pubchem.ncbi.nlm.nih.gov | |
Description | Data deposited in or computed by PubChem | |
Canonical SMILES |
CCCCCCCCOC1=CC=CC=C1C2=CC(=CC(=N2)C3=CC=CC=C3)C4=CC=C(C=C4)N(C)C |
Source
|
Source | PubChem | |
URL | https://pubchem.ncbi.nlm.nih.gov | |
Description | Data deposited in or computed by PubChem | |
Molecular Formula |
C33H38N2O |
Source
|
Source | PubChem | |
URL | https://pubchem.ncbi.nlm.nih.gov | |
Description | Data deposited in or computed by PubChem | |
DSSTOX Substance ID |
DTXSID30596007 |
Source
|
Record name | N,N-Dimethyl-4-{2-[2-(octyloxy)phenyl]-6-phenylpyridin-4-yl}aniline | |
Source | EPA DSSTox | |
URL | https://comptox.epa.gov/dashboard/DTXSID30596007 | |
Description | DSSTox provides a high quality public chemistry resource for supporting improved predictive toxicology. | |
Molecular Weight |
478.7 g/mol |
Source
|
Source | PubChem | |
URL | https://pubchem.ncbi.nlm.nih.gov | |
Description | Data deposited in or computed by PubChem | |
CAS No. |
144190-25-0 |
Source
|
Record name | N,N-Dimethyl-4-{2-[2-(octyloxy)phenyl]-6-phenylpyridin-4-yl}aniline | |
Source | EPA DSSTox | |
URL | https://comptox.epa.gov/dashboard/DTXSID30596007 | |
Description | DSSTox provides a high quality public chemistry resource for supporting improved predictive toxicology. | |
体外研究产品的免责声明和信息
请注意,BenchChem 上展示的所有文章和产品信息仅供信息参考。 BenchChem 上可购买的产品专为体外研究设计,这些研究在生物体外进行。体外研究,源自拉丁语 "in glass",涉及在受控实验室环境中使用细胞或组织进行的实验。重要的是要注意,这些产品没有被归类为药物或药品,他们没有得到 FDA 的批准,用于预防、治疗或治愈任何医疗状况、疾病或疾病。我们必须强调,将这些产品以任何形式引入人类或动物的身体都是法律严格禁止的。遵守这些指南对确保研究和实验的法律和道德标准的符合性至关重要。
快速询问
类别
最多查看
10-Piperazinylpropylphenothiazine
Cat. No.: B130854
CAS No.: 3240-48-0
3-(cyclopropylmethoxy)-4-(difluoromethoxy)benzoic Acid
Cat. No.: B130855
CAS No.: 162401-62-9
(S)-Neolitsine
Cat. No.: B130865
CAS No.: 2466-42-4
5-Propynylcytosine
Cat. No.: B130867
CAS No.: 151091-68-8