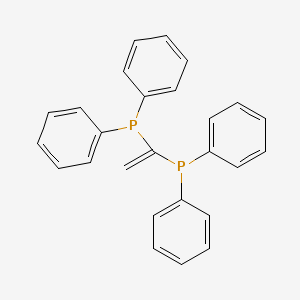
1,1-Bis(diphenylphosphino)ethylene
概述
描述
1,1-Bis(diphenylphosphino)ethylene is an organophosphorus compound with the chemical formula ([(C_6H_5)_2P]_2C=CH_2). It is commonly used as a ligand in coordination chemistry due to its ability to form stable complexes with transition metals. This compound is known for its versatility and effectiveness in various catalytic processes.
作用机制
Target of Action
1,1-Bis(diphenylphosphino)ethylene, also known as Ethene-1,1-diylbis(diphenylphosphine), is primarily used as a ligand in the field of organometallic chemistry . It targets metal centers, stabilizing them through chelation . This compound is particularly useful in transition metal-catalyzed transformations .
Mode of Action
The compound acts by binding to metal centers in a bidentate manner, meaning it attaches at two points . This mode of action is facilitated by the presence of lone pair electrons on the phosphorus atoms, which have a certain nucleophilicity and can form coordinate bonds with metal centers .
Biochemical Pathways
For instance, it is used as a reactant for the preparation of cyclometallated palladium diphosphane catalysts, functionalized bis-phosphine monoxide ligands, and tetranuclear palladacycles . It is also used in chromium-catalyzed ethylene tri- and tetramerization reactions .
Result of Action
The action of this compound results in the formation of stable metal complexes that can act as catalysts in various chemical reactions . For example, it can facilitate the conversion of terminal alkynes into propiolic acids with CO2 .
生化分析
Biochemical Properties
1,1-Bis(diphenylphosphino)ethylene plays a significant role in biochemical reactions as a ligand. It interacts with various enzymes and proteins, particularly those involved in metal-catalyzed reactions. For example, it forms complexes with palladium, copper, and nickel, which are used in catalytic processes such as Michael addition reactions and ethylene tri- and tetramerization reactions . The nature of these interactions involves the coordination of the phosphorus atoms in this compound with the metal centers, stabilizing the metal complexes and enhancing their catalytic activity.
Cellular Effects
The effects of this compound on various types of cells and cellular processes are primarily related to its role as a ligand in metal-catalyzed reactions. It influences cell function by facilitating catalytic processes that are essential for cellular metabolism and gene expression. For instance, the formation of metal complexes with this compound can impact cell signaling pathways and enzymatic activities, thereby affecting cellular metabolism and gene expression .
Molecular Mechanism
At the molecular level, this compound exerts its effects through binding interactions with metal centers in enzymes and proteins. These interactions involve the coordination of the phosphorus atoms in this compound with the metal ions, leading to the formation of stable metal-ligand complexes. These complexes can either inhibit or activate enzymatic activities, depending on the specific metal and reaction involved . Additionally, this compound can influence gene expression by modulating the activity of metal-dependent transcription factors.
Temporal Effects in Laboratory Settings
In laboratory settings, the effects of this compound can change over time due to its stability and degradation. The compound is generally stable under standard laboratory conditions, but its long-term effects on cellular function can vary depending on the specific experimental setup. Studies have shown that this compound can maintain its catalytic activity over extended periods, although degradation products may form under certain conditions .
Dosage Effects in Animal Models
The effects of this compound in animal models vary with different dosages. At low doses, the compound can enhance catalytic processes and improve metabolic functions. At high doses, it may exhibit toxic or adverse effects, such as respiratory irritation and damage to the respiratory tract . Threshold effects have been observed, indicating that there is a specific dosage range within which this compound is effective without causing significant toxicity.
Metabolic Pathways
This compound is involved in various metabolic pathways, particularly those related to metal-catalyzed reactions. It interacts with enzymes and cofactors that facilitate these reactions, influencing metabolic flux and metabolite levels. For example, the compound can participate in the catalytic conversion of ethylene to higher hydrocarbons, a process that involves multiple enzymatic steps and cofactors .
Transport and Distribution
Within cells and tissues, this compound is transported and distributed through interactions with transporters and binding proteins. These interactions can affect the localization and accumulation of the compound, influencing its activity and function. The compound’s ability to form stable metal complexes also plays a role in its transport and distribution, as these complexes can be selectively transported to specific cellular compartments .
Subcellular Localization
The subcellular localization of this compound is influenced by targeting signals and post-translational modifications that direct it to specific compartments or organelles. For instance, the compound may be localized to the mitochondria or endoplasmic reticulum, where it can participate in metal-catalyzed reactions essential for cellular metabolism . The localization of this compound can also affect its activity and function, as the formation of metal complexes in specific subcellular compartments can enhance or inhibit enzymatic activities.
准备方法
Synthetic Routes and Reaction Conditions: 1,1-Bis(diphenylphosphino)ethylene can be synthesized through the reaction of lithium diphenylphosphide with dichloroethylene. The reaction typically proceeds as follows: [ 2 \text{LiPPh}_2 + \text{C}_2\text{H}_2\text{Cl}_2 \rightarrow \text{C}_2\text{H}_2(\text{PPh}_2)_2 + 2 \text{LiCl} ] This method involves the use of lithium diphenylphosphide as a nucleophile, which reacts with dichloroethylene to form the desired product .
Industrial Production Methods: Industrial production of this compound follows similar synthetic routes but on a larger scale. The reaction conditions are optimized to ensure high yield and purity of the product. The use of advanced purification techniques, such as recrystallization and chromatography, is common to achieve the desired quality.
化学反应分析
1,1-Bis(diphenylphosphino)ethylene undergoes various types of chemical reactions, including:
Oxidation: The compound can be oxidized to form phosphine oxides. Common oxidizing agents include hydrogen peroxide and other peroxides.
Reduction: Reduction reactions typically involve the use of reducing agents like lithium aluminum hydride (LiAlH_4) to convert the compound into its reduced form.
Substitution: this compound can participate in nucleophilic substitution reactions, where the phosphine groups act as nucleophiles. Common reagents include alkyl halides and acyl chlorides.
Major Products: The major products formed from these reactions depend on the specific conditions and reagents used. For example, oxidation typically yields phosphine oxides, while substitution reactions can produce various phosphine derivatives .
科学研究应用
1,1-Bis(diphenylphosphino)ethylene has a wide range of applications in scientific research:
Chemistry:
- Used as a ligand in the synthesis of coordination complexes with transition metals.
- Employed in catalytic processes, such as hydrogenation and carbonylation reactions.
Biology:
- Investigated for its potential use in biological systems as a ligand for metal-based drugs.
Medicine:
- Explored for its role in the development of new pharmaceuticals, particularly those involving metal coordination complexes.
Industry:
- Utilized in the production of fine chemicals and specialty materials.
- Applied in the development of new catalysts for industrial processes .
相似化合物的比较
1,1-Bis(diphenylphosphino)ethylene can be compared with other similar compounds, such as:
1,2-Bis(diphenylphosphino)ethylene:
- Similar in structure but differs in the position of the phosphine groups.
- Both compounds are used as ligands, but their coordination properties and reactivity can vary.
Ethylenebis(diphenylphosphine):
- Another related compound with a different arrangement of the phosphine groups.
- Used in similar applications but may exhibit different catalytic properties .
These comparisons highlight the uniqueness of this compound in terms of its structure and reactivity, making it a valuable compound in various fields of research and industry.
属性
IUPAC Name |
1-diphenylphosphanylethenyl(diphenyl)phosphane | |
---|---|---|
Source | PubChem | |
URL | https://pubchem.ncbi.nlm.nih.gov | |
Description | Data deposited in or computed by PubChem | |
InChI |
InChI=1S/C26H22P2/c1-22(27(23-14-6-2-7-15-23)24-16-8-3-9-17-24)28(25-18-10-4-11-19-25)26-20-12-5-13-21-26/h2-21H,1H2 | |
Source | PubChem | |
URL | https://pubchem.ncbi.nlm.nih.gov | |
Description | Data deposited in or computed by PubChem | |
InChI Key |
GEGLBMPXRFOXTK-UHFFFAOYSA-N | |
Source | PubChem | |
URL | https://pubchem.ncbi.nlm.nih.gov | |
Description | Data deposited in or computed by PubChem | |
Canonical SMILES |
C=C(P(C1=CC=CC=C1)C2=CC=CC=C2)P(C3=CC=CC=C3)C4=CC=CC=C4 | |
Source | PubChem | |
URL | https://pubchem.ncbi.nlm.nih.gov | |
Description | Data deposited in or computed by PubChem | |
Molecular Formula |
C26H22P2 | |
Source | PubChem | |
URL | https://pubchem.ncbi.nlm.nih.gov | |
Description | Data deposited in or computed by PubChem | |
DSSTOX Substance ID |
DTXSID20401979 | |
Record name | 1,1-Bis(diphenylphosphino)ethylene | |
Source | EPA DSSTox | |
URL | https://comptox.epa.gov/dashboard/DTXSID20401979 | |
Description | DSSTox provides a high quality public chemistry resource for supporting improved predictive toxicology. | |
Molecular Weight |
396.4 g/mol | |
Source | PubChem | |
URL | https://pubchem.ncbi.nlm.nih.gov | |
Description | Data deposited in or computed by PubChem | |
CAS No. |
84494-89-3 | |
Record name | 1,1-Bis(diphenylphosphino)ethylene | |
Source | EPA DSSTox | |
URL | https://comptox.epa.gov/dashboard/DTXSID20401979 | |
Description | DSSTox provides a high quality public chemistry resource for supporting improved predictive toxicology. | |
Record name | 1,1-Bis(diphenylphosphino)ethylene | |
Source | European Chemicals Agency (ECHA) | |
URL | https://echa.europa.eu/information-on-chemicals | |
Description | The European Chemicals Agency (ECHA) is an agency of the European Union which is the driving force among regulatory authorities in implementing the EU's groundbreaking chemicals legislation for the benefit of human health and the environment as well as for innovation and competitiveness. | |
Explanation | Use of the information, documents and data from the ECHA website is subject to the terms and conditions of this Legal Notice, and subject to other binding limitations provided for under applicable law, the information, documents and data made available on the ECHA website may be reproduced, distributed and/or used, totally or in part, for non-commercial purposes provided that ECHA is acknowledged as the source: "Source: European Chemicals Agency, http://echa.europa.eu/". Such acknowledgement must be included in each copy of the material. ECHA permits and encourages organisations and individuals to create links to the ECHA website under the following cumulative conditions: Links can only be made to webpages that provide a link to the Legal Notice page. | |
Synthesis routes and methods
Procedure details
Retrosynthesis Analysis
AI-Powered Synthesis Planning: Our tool employs the Template_relevance Pistachio, Template_relevance Bkms_metabolic, Template_relevance Pistachio_ringbreaker, Template_relevance Reaxys, Template_relevance Reaxys_biocatalysis model, leveraging a vast database of chemical reactions to predict feasible synthetic routes.
One-Step Synthesis Focus: Specifically designed for one-step synthesis, it provides concise and direct routes for your target compounds, streamlining the synthesis process.
Accurate Predictions: Utilizing the extensive PISTACHIO, BKMS_METABOLIC, PISTACHIO_RINGBREAKER, REAXYS, REAXYS_BIOCATALYSIS database, our tool offers high-accuracy predictions, reflecting the latest in chemical research and data.
Strategy Settings
Precursor scoring | Relevance Heuristic |
---|---|
Min. plausibility | 0.01 |
Model | Template_relevance |
Template Set | Pistachio/Bkms_metabolic/Pistachio_ringbreaker/Reaxys/Reaxys_biocatalysis |
Top-N result to add to graph | 6 |
Feasible Synthetic Routes
Q1: How does dppen interact with metal centers, and what structural motifs are commonly observed?
A1: Dppen readily coordinates to various transition metals, typically acting as a bidentate ligand through its two phosphorus atoms. This coordination forms a five-membered chelate ring with the metal center. [, ] For instance, in [Fe2(CO)7{µ-(Ph2P)2CCH2}] and [Fe3(CO)10{µ-(Ph2P)2CCH2}], dppen bridges two iron centers. [] Interestingly, under specific conditions, dppen can undergo fragmentation, leading to the formation of μ3-vinylidene complexes as seen in the formation of [Fe3(CO)6(µ-CO)(µ-PPh2)2(µ3-CCH2)].[1] This highlights the potential for dppen to act as a precursor for other valuable ligands in organometallic synthesis.
Q2: Can you elaborate on the role of dppen in the development of catalytic systems, particularly for upgrading ethanol and methanol?
A2: Dppen-containing ruthenium complexes have shown promising catalytic activity in the Guerbet-type upgrading of ethanol and methanol to iso-butanol. [] This reaction is particularly important for generating higher-order alcohols from renewable sources. The backbone functionalization of dppen with amine groups via Michael addition has been shown to enhance catalytic performance. [] For example, catalyst 1, containing a functionalized dppen ligand, demonstrated a 74% yield of iso-butanol in just 2 hours. [] This example highlights the importance of ligand modification in tuning catalyst activity and selectivity.
Q3: What analytical techniques are commonly employed to characterize dppen-containing metal complexes?
A3: Characterization of dppen-containing complexes relies heavily on a combination of spectroscopic and crystallographic techniques. Single-crystal X-ray diffraction (SC-XRD) is frequently used to elucidate the solid-state structures of these complexes, revealing bond lengths, angles, and coordination geometries. [, ] Electrospray ionization mass spectrometry (ESI-MS) is another valuable tool for confirming the composition of synthesized complexes, particularly when crystal growth is challenging. [] Additionally, nuclear magnetic resonance (NMR) spectroscopy, particularly phosphorus-31 NMR, provides insights into the coordination environment of the dppen ligand and its interaction with the metal center.
体外研究产品的免责声明和信息
请注意,BenchChem 上展示的所有文章和产品信息仅供信息参考。 BenchChem 上可购买的产品专为体外研究设计,这些研究在生物体外进行。体外研究,源自拉丁语 "in glass",涉及在受控实验室环境中使用细胞或组织进行的实验。重要的是要注意,这些产品没有被归类为药物或药品,他们没有得到 FDA 的批准,用于预防、治疗或治愈任何医疗状况、疾病或疾病。我们必须强调,将这些产品以任何形式引入人类或动物的身体都是法律严格禁止的。遵守这些指南对确保研究和实验的法律和道德标准的符合性至关重要。