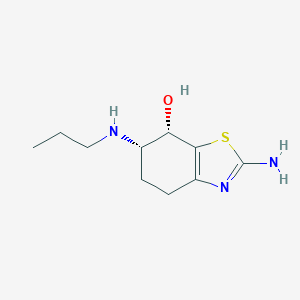
(6S,7S)-2-氨基-4,5,6,7-四氢-6-(丙基氨基)-7-苯并噻唑
- 点击 快速询问 获取最新报价。
- 提供有竞争力价格的高质量产品,您可以更专注于研究。
描述
(6S,7S)-2-amino-6-(propylamino)-4,5,6,7-tetrahydro-1,3-benzothiazol-7-ol, also known as (6S,7S)-2-amino-6-(propylamino)-4,5,6,7-tetrahydro-1,3-benzothiazol-7-ol, is a useful research compound. Its molecular formula is C10H17N3OS and its molecular weight is 227.33 g/mol. The purity is usually 95%.
BenchChem offers high-quality (6S,7S)-2-amino-6-(propylamino)-4,5,6,7-tetrahydro-1,3-benzothiazol-7-ol suitable for many research applications. Different packaging options are available to accommodate customers' requirements. Please inquire for more information about (6S,7S)-2-amino-6-(propylamino)-4,5,6,7-tetrahydro-1,3-benzothiazol-7-ol including the price, delivery time, and more detailed information at info@benchchem.com.
科学研究应用
企业社会责任 (CSR) 整合 (7S)
在 6S 的基础上,7S 方法旨在促进将 CSR 政策纳入组织。以下是你需要了解的信息:
普拉克索相关化合物 G
有趣的是,该化合物 (6S,7S)-2-氨基-4,5,6,7-四氢-6-(丙基氨基)-7-苯并噻唑 也与 普拉克索 相关。普拉克索是一种用于治疗帕金森病和不安腿综合征的药物。 你指定的化合物可能在这些治疗领域有影响,尽管需要进一步的研究来探索其具体应用 .
属性
IUPAC Name |
(6S,7S)-2-amino-6-(propylamino)-4,5,6,7-tetrahydro-1,3-benzothiazol-7-ol |
Source
|
---|---|---|
Source | PubChem | |
URL | https://pubchem.ncbi.nlm.nih.gov | |
Description | Data deposited in or computed by PubChem | |
InChI |
InChI=1S/C10H17N3OS/c1-2-5-12-6-3-4-7-9(8(6)14)15-10(11)13-7/h6,8,12,14H,2-5H2,1H3,(H2,11,13)/t6-,8-/m0/s1 |
Source
|
Source | PubChem | |
URL | https://pubchem.ncbi.nlm.nih.gov | |
Description | Data deposited in or computed by PubChem | |
InChI Key |
UWNDKVURLHPSSG-XPUUQOCRSA-N |
Source
|
Source | PubChem | |
URL | https://pubchem.ncbi.nlm.nih.gov | |
Description | Data deposited in or computed by PubChem | |
Canonical SMILES |
CCCNC1CCC2=C(C1O)SC(=N2)N |
Source
|
Source | PubChem | |
URL | https://pubchem.ncbi.nlm.nih.gov | |
Description | Data deposited in or computed by PubChem | |
Isomeric SMILES |
CCCN[C@H]1CCC2=C([C@H]1O)SC(=N2)N |
Source
|
Source | PubChem | |
URL | https://pubchem.ncbi.nlm.nih.gov | |
Description | Data deposited in or computed by PubChem | |
Molecular Formula |
C10H17N3OS |
Source
|
Source | PubChem | |
URL | https://pubchem.ncbi.nlm.nih.gov | |
Description | Data deposited in or computed by PubChem | |
DSSTOX Substance ID |
DTXSID20648044 |
Source
|
Record name | (6S,7S)-2-Amino-6-(propylamino)-4,5,6,7-tetrahydro-1,3-benzothiazol-7-ol | |
Source | EPA DSSTox | |
URL | https://comptox.epa.gov/dashboard/DTXSID20648044 | |
Description | DSSTox provides a high quality public chemistry resource for supporting improved predictive toxicology. | |
Molecular Weight |
227.33 g/mol |
Source
|
Source | PubChem | |
URL | https://pubchem.ncbi.nlm.nih.gov | |
Description | Data deposited in or computed by PubChem | |
CAS No. |
1001648-71-0 |
Source
|
Record name | (6S,7S)-2-Amino-4,5,6,7-tetrahydro-6-(propylamino)-7-benzothiazolol | |
Source | ChemIDplus | |
URL | https://pubchem.ncbi.nlm.nih.gov/substance/?source=chemidplus&sourceid=1001648710 | |
Description | ChemIDplus is a free, web search system that provides access to the structure and nomenclature authority files used for the identification of chemical substances cited in National Library of Medicine (NLM) databases, including the TOXNET system. | |
Record name | (6S,7S)-2-Amino-6-(propylamino)-4,5,6,7-tetrahydro-1,3-benzothiazol-7-ol | |
Source | EPA DSSTox | |
URL | https://comptox.epa.gov/dashboard/DTXSID20648044 | |
Description | DSSTox provides a high quality public chemistry resource for supporting improved predictive toxicology. | |
Record name | (6S,7S)-2-AMINO-4,5,6,7-TETRAHYDRO-6-(PROPYLAMINO)-7-BENZOTHIAZOLOL | |
Source | FDA Global Substance Registration System (GSRS) | |
URL | https://gsrs.ncats.nih.gov/ginas/app/beta/substances/W5S8JXQ9VB | |
Description | The FDA Global Substance Registration System (GSRS) enables the efficient and accurate exchange of information on what substances are in regulated products. Instead of relying on names, which vary across regulatory domains, countries, and regions, the GSRS knowledge base makes it possible for substances to be defined by standardized, scientific descriptions. | |
Explanation | Unless otherwise noted, the contents of the FDA website (www.fda.gov), both text and graphics, are not copyrighted. They are in the public domain and may be republished, reprinted and otherwise used freely by anyone without the need to obtain permission from FDA. Credit to the U.S. Food and Drug Administration as the source is appreciated but not required. | |
体外研究产品的免责声明和信息
请注意,BenchChem 上展示的所有文章和产品信息仅供信息参考。 BenchChem 上可购买的产品专为体外研究设计,这些研究在生物体外进行。体外研究,源自拉丁语 "in glass",涉及在受控实验室环境中使用细胞或组织进行的实验。重要的是要注意,这些产品没有被归类为药物或药品,他们没有得到 FDA 的批准,用于预防、治疗或治愈任何医疗状况、疾病或疾病。我们必须强调,将这些产品以任何形式引入人类或动物的身体都是法律严格禁止的。遵守这些指南对确保研究和实验的法律和道德标准的符合性至关重要。