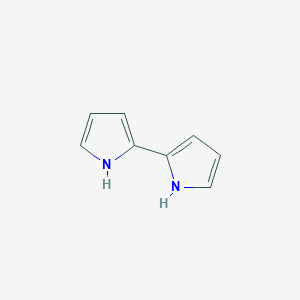
2,2'-联吡咯
描述
2,2’-Bipyrrole is a chemical compound with the molecular formula C8H8N2 . It is a basic building block for the preparation of pyrrolic macrocycles and natural products, such as prodigiosines .
Synthesis Analysis
Functionalized N-unsubstituted 2,2’-bipyrroles are used in the preparation of pyrrolic macrocycles and natural products . The synthesis of 2,2’-bipyrroles substituted at positions 5,5’ with pyrrolyl, N-methyl-pyrrolyl, and thienyl groups is reported, and these monomers can be polymerized through oxidative/electropolymerization .
Molecular Structure Analysis
The complete molecule of 2,2’-Bipyrrole is generated by a crystallographic center of symmetry . The pyrrole rings are exactly parallel and the N—H groups face in opposite directions .
Chemical Reactions Analysis
Functionalized N-unsubstituted 2,2’-bipyrroles are basic building blocks for the preparation of pyrrolic macrocycles and natural products . They play a central role as building blocks for the synthesis of porphyrinoids and property-defining structural elements therein .
Physical And Chemical Properties Analysis
2,2’-Bipyrrole has a density of 1.2±0.1 g/cm3, a boiling point of 372.1±17.0 °C at 760 mmHg, and a vapour pressure of 0.0±0.8 mmHg at 25°C . It has an enthalpy of vaporization of 59.5±3.0 kJ/mol and a flash point of 177.0±12.2 °C . The index of refraction is 1.632, and it has a molar refractivity of 39.7±0.3 cm3 .
科学研究应用
Iron Detection in Soil
2,2’-Bipyrrole is utilized for the colorimetric determination of iron, particularly to confirm the presence of ferrous ion in soils. This application is crucial in agronomy and environmental science for assessing soil health and fertility .
Luminescent Complexes
This compound forms complexes with metals like ruthenium and platinum, which exhibit intense luminescence. Such luminescent properties have potential practical applications in areas like light-emitting devices and sensors .
Synthesis of Porphyrinoids
2,2’-Bipyrrole serves as a building block for the synthesis of porphyrinoids, which are significant in fields like biochemistry and materials science due to their property-defining structural elements .
Conjugated Polymers
Extended 2,2’-Bipyrroles are used as new monomers for conjugated polymers, which have applications in organic electronics due to their low oxidation potential and extended conjugation pathways .
Electrochemical Characterization
The electrochemical properties of 2,2’-Bipyrrole monomers are characterized for their potential use in electronic devices. Electropolymerization studies are conducted to explore their suitability for various electronic applications .
Oxidation-Reduction Indicators
In analytical chemistry, 2,2’-Bipyrrole is used as an oxidation-reduction indicator due to its ability to undergo reversible redox reactions, which is essential for studying electron transfer processes .
安全和危害
作用机制
Target of Action
2,2’-Bipyrrole is a basic building block for the preparation of pyrrolic macrocycles and natural products, such as prodigiosines .
Mode of Action
It is known that the compound has a low oxidation potential due to the extended length of its conjugation pathway , which suggests it may interact with its targets through redox reactions
Biochemical Pathways
2,2’-Bipyrrole is involved in the synthesis of porphyrinoids and other property-defining structural elements . It is also a possible common biosynthetic intermediate of pyrrole-containing natural products including prodigiosins and tambjamines . .
Result of Action
One study found that 4-methoxy-2,2’-bipyrrole-5-carbaldehyde (MBC), a possible biosynthetic intermediate of 2,2’-Bipyrrole, arrested the strobilation of moon jellyfish Aurelia coerulea without inducing cytotoxicity and generated abnormal tentacle-like structures in a dose-dependent manner .
属性
IUPAC Name |
2-(1H-pyrrol-2-yl)-1H-pyrrole | |
---|---|---|
Source | PubChem | |
URL | https://pubchem.ncbi.nlm.nih.gov | |
Description | Data deposited in or computed by PubChem | |
InChI |
InChI=1S/C8H8N2/c1-3-7(9-5-1)8-4-2-6-10-8/h1-6,9-10H | |
Source | PubChem | |
URL | https://pubchem.ncbi.nlm.nih.gov | |
Description | Data deposited in or computed by PubChem | |
InChI Key |
ULUNQYODBKLBOE-UHFFFAOYSA-N | |
Source | PubChem | |
URL | https://pubchem.ncbi.nlm.nih.gov | |
Description | Data deposited in or computed by PubChem | |
Canonical SMILES |
C1=CNC(=C1)C2=CC=CN2 | |
Source | PubChem | |
URL | https://pubchem.ncbi.nlm.nih.gov | |
Description | Data deposited in or computed by PubChem | |
Molecular Formula |
C8H8N2 | |
Source | PubChem | |
URL | https://pubchem.ncbi.nlm.nih.gov | |
Description | Data deposited in or computed by PubChem | |
DSSTOX Substance ID |
DTXSID40293595 | |
Record name | 2,2'-Bipyrrole | |
Source | EPA DSSTox | |
URL | https://comptox.epa.gov/dashboard/DTXSID40293595 | |
Description | DSSTox provides a high quality public chemistry resource for supporting improved predictive toxicology. | |
Molecular Weight |
132.16 g/mol | |
Source | PubChem | |
URL | https://pubchem.ncbi.nlm.nih.gov | |
Description | Data deposited in or computed by PubChem | |
Product Name |
2,2'-Bipyrrole | |
CAS RN |
10087-64-6 | |
Record name | 2,2'-Bipyrrole | |
Source | DTP/NCI | |
URL | https://dtp.cancer.gov/dtpstandard/servlet/dwindex?searchtype=NSC&outputformat=html&searchlist=90886 | |
Description | The NCI Development Therapeutics Program (DTP) provides services and resources to the academic and private-sector research communities worldwide to facilitate the discovery and development of new cancer therapeutic agents. | |
Explanation | Unless otherwise indicated, all text within NCI products is free of copyright and may be reused without our permission. Credit the National Cancer Institute as the source. | |
Record name | 2,2'-Bipyrrole | |
Source | EPA DSSTox | |
URL | https://comptox.epa.gov/dashboard/DTXSID40293595 | |
Description | DSSTox provides a high quality public chemistry resource for supporting improved predictive toxicology. | |
Retrosynthesis Analysis
AI-Powered Synthesis Planning: Our tool employs the Template_relevance Pistachio, Template_relevance Bkms_metabolic, Template_relevance Pistachio_ringbreaker, Template_relevance Reaxys, Template_relevance Reaxys_biocatalysis model, leveraging a vast database of chemical reactions to predict feasible synthetic routes.
One-Step Synthesis Focus: Specifically designed for one-step synthesis, it provides concise and direct routes for your target compounds, streamlining the synthesis process.
Accurate Predictions: Utilizing the extensive PISTACHIO, BKMS_METABOLIC, PISTACHIO_RINGBREAKER, REAXYS, REAXYS_BIOCATALYSIS database, our tool offers high-accuracy predictions, reflecting the latest in chemical research and data.
Strategy Settings
Precursor scoring | Relevance Heuristic |
---|---|
Min. plausibility | 0.01 |
Model | Template_relevance |
Template Set | Pistachio/Bkms_metabolic/Pistachio_ringbreaker/Reaxys/Reaxys_biocatalysis |
Top-N result to add to graph | 6 |
Feasible Synthetic Routes
Q & A
Q1: What is the molecular formula and weight of 2,2'-bipyrrole?
A1: The molecular formula of 2,2'-bipyrrole is C8H8N2, and its molecular weight is 132.16 g/mol.
Q2: How does the conformation of 2,2'-bipyrrole affect its properties?
A2: 2,2'-Bipyrrole can exist in cis and trans conformations. The conformation affects its electronic properties, reactivity, and ability to form complexes. For example, [studies on 1,1',5,5'-tetraaryl-2,2'-bipyrroles revealed that the conformation between the two pyrrole rings, influenced by substituents, impacts their UV-VIS spectra] [].
Q3: What spectroscopic techniques are used to characterize 2,2'-bipyrrole and its derivatives?
A3: Common techniques include UV-Vis absorption, fluorescence, IR spectroscopy, NMR spectroscopy, and cyclic voltammetry. These methods help determine structure, electronic properties, and redox behavior. For instance, [researchers employed UV-VIS absorption and fluorescence spectroscopy to investigate the conformations of 2,2′-bipyrrole π-systems] [].
Q4: What are the common synthetic routes for preparing 2,2'-bipyrroles?
A4: Several methods exist, including Pd(0)-catalyzed homocoupling of 2-iodopyrroles [, ], oxidative coupling of pyrroles [, , ], and reactions involving donor-acceptor cyclopropanes with 2-cyanopyrroles [].
Q5: How can the solubility of 2,2'-bipyrroles be tailored?
A5: Solubility can be modified by introducing various substituents on the pyrrole rings. [Research has shown that the solubility of 2,2′-bisdipyrrins, derivatives of 2,2'-bipyrrole, can be easily tuned by modifying the peripheral substituent pattern] [].
Q6: How do substituents affect the reactivity of 2,2'-bipyrroles?
A6: Substituents significantly influence the electronic properties and, therefore, the reactivity of 2,2'-bipyrroles. For instance, [introducing electron-donating methoxy groups at the 3,3' or 4,4' positions of 2,2'-bipyrrole results in highly electron-rich compounds with low oxidation potentials] [].
Q7: What is the significance of 2,2'-bipyrrole in the synthesis of expanded porphyrins?
A7: 2,2'-Bipyrrole serves as a crucial building block for synthesizing diverse porphyrinoids, including larger macrocycles like octaphyrins [, , , , ] and cyclo[8]pyrroles [, ].
Q8: How do the properties of 2,2'-bipyrrole-based porphyrinoids differ from traditional porphyrins?
A8: The incorporation of 2,2'-bipyrrole units into porphyrinoids introduces unique features. These include modified cavity shapes, distinct electronic properties due to cyclo-π-conjugation [], and potential for anion binding [, ].
Q9: What is the biological significance of 2,2'-bipyrrole?
A9: 2,2'-Bipyrrole is a key structural element in prodigiosins, a family of tripyrrole natural products with reported anticancer, antimicrobial, and immunosuppressive activities [, , , , ].
Q10: How does the structure of prodigiosins contribute to their biological activity?
A10: The 4-methoxy-2,2'-bipyrrole core is essential for DNA binding and biological activity. The enamine and pyrromethene moieties in different prodigiosins influence their binding affinity and selectivity [, ].
Q11: What is known about the biosynthesis of prodigiosin?
A11: Prodigiosin biosynthesis involves a bifurcated pathway where 4-methoxy-2,2'-bipyrrole-5-carboxaldehyde (MBC) and 2-methyl-3-amylpyrrole (MAP) are synthesized separately and then condensed [, , ]. Enzymes like PigF and RedH play crucial roles in this pathway [, ].
Q12: How is computational chemistry used in research on 2,2'-bipyrrole and its derivatives?
A12: Computational tools aid in understanding conformational preferences, electronic structures, and interactions with other molecules. For instance, [theoretical calculations have been employed to study the twist angles, torsional potentials, and electronic transitions in 2,2'-bipyrrole and its benzo-fused analogs] [].
体外研究产品的免责声明和信息
请注意,BenchChem 上展示的所有文章和产品信息仅供信息参考。 BenchChem 上可购买的产品专为体外研究设计,这些研究在生物体外进行。体外研究,源自拉丁语 "in glass",涉及在受控实验室环境中使用细胞或组织进行的实验。重要的是要注意,这些产品没有被归类为药物或药品,他们没有得到 FDA 的批准,用于预防、治疗或治愈任何医疗状况、疾病或疾病。我们必须强调,将这些产品以任何形式引入人类或动物的身体都是法律严格禁止的。遵守这些指南对确保研究和实验的法律和道德标准的符合性至关重要。