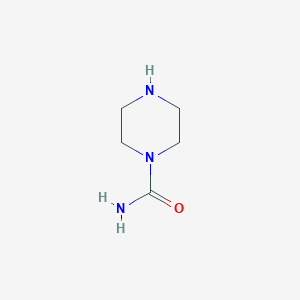
Piperazine-1-carboxamide
概述
描述
Piperazine-1-carboxamide is a chemical compound that belongs to the class of piperazine derivatives. Piperazine itself is a heterocyclic organic compound that consists of a six-membered ring containing two nitrogen atoms at opposite positions. This compound is characterized by the presence of a carboxamide group (-CONH2) attached to the piperazine ring. This compound is of significant interest due to its diverse applications in medicinal chemistry, particularly in the development of pharmaceutical agents.
作用机制
Target of Action
Piperazine-1-carboxamide has been identified as a potent inhibitor of the enzyme Fatty Acid Amide Hydrolase (FAAH) . FAAH is an integral membrane protein responsible for the breakdown of fatty acid amides, including anandamide, a neurotransmitter involved in pain sensation, mood, and memory .
Mode of Action
This compound interacts with FAAH by binding to its active site, thereby inhibiting the enzyme’s activity . This inhibition prevents the breakdown of anandamide, leading to an increase in its concentration in the brain . As a result, the effects of anandamide are enhanced, which can lead to reduced pain sensation and improved mood .
Biochemical Pathways
The primary biochemical pathway affected by this compound is the endocannabinoid system . This system is involved in a variety of physiological processes, including pain sensation, mood, and memory. By inhibiting FAAH, this compound increases the concentration of anandamide, a key endocannabinoid, leading to enhanced signaling in this pathway .
Result of Action
The primary result of this compound’s action is an increase in anandamide levels in the brain, leading to enhanced endocannabinoid signaling . This can result in a variety of effects, depending on the specific physiological processes influenced by the endocannabinoid system. For example, increased anandamide levels can lead to reduced pain sensation and improved mood .
Action Environment
The action of this compound, like many drugs, can be influenced by a variety of environmental factors. For instance, the pH of the environment can affect the drug’s stability and solubility, potentially impacting its absorption and distribution . Additionally, factors such as temperature and the presence of other substances can influence the drug’s stability and efficacy . .
生化分析
Biochemical Properties
Piperazine-1-carboxamide plays a significant role in biochemical reactions due to its ability to interact with various enzymes, proteins, and other biomolecules. One of the key interactions is with the enzyme fatty acid amide hydrolase (FAAH), where this compound acts as an inhibitor . This inhibition can modulate the levels of fatty acid amides, which are involved in various physiological processes such as pain, inflammation, and mood regulation.
Additionally, this compound has been shown to interact with the enzyme acetylcholinesterase (AchE), inhibiting its activity . This interaction affects the breakdown of acetylcholine, a neurotransmitter, thereby influencing neuromuscular and cholinergic brain synapses.
Cellular Effects
This compound has been observed to exert various effects on different types of cells and cellular processes. In cancer cells, it has been shown to induce apoptosis, a programmed cell death mechanism, by upregulating intracellular apoptotic marker proteins such as cleaved caspase-3, cytochrome c, and Bax . This compound also increases the activities of caspase-3 and -9, which are crucial for the execution of apoptosis.
Furthermore, this compound influences cell signaling pathways, particularly the TEA domain transcription factor (TEAD)-YAP pathway, which is involved in cell proliferation, development, and tumorigenesis . By inhibiting the TEAD-YAP complex, this compound can suppress the transcriptional activities of genes responsive to the Hippo pathway, leading to reduced cancer cell proliferation and survival.
Molecular Mechanism
The molecular mechanism of this compound involves several binding interactions with biomolecules. One notable interaction is with the palmitate-binding pocket (PBP) of TEAD transcription factors, where this compound acts as an inhibitor . This binding inhibits the auto-palmitoylation activity of TEAD, thereby modulating its function in gene expression.
This compound also interacts with mitochondrial fission machinery in yeast cells, inducing caspase-dependent apoptosis . This interaction involves proteins such as Fis1, Dnm1, and Mdv1, which play roles in mitochondrial fission and apoptosis.
Temporal Effects in Laboratory Settings
In laboratory settings, the effects of this compound can change over time due to its stability and degradation. Studies have shown that this compound is relatively stable under controlled conditions, but its degradation can occur over extended periods . Long-term effects on cellular function have been observed in both in vitro and in vivo studies, with sustained inhibition of target enzymes and prolonged apoptotic effects in cancer cells.
Dosage Effects in Animal Models
The effects of this compound vary with different dosages in animal models. At lower doses, it has been shown to effectively inhibit FAAH and AchE, leading to reduced pain and inflammation . At higher doses, this compound can cause neurotoxic symptoms such as tremors, convulsions, and CNS depression in animals . These adverse effects highlight the importance of determining the optimal dosage for therapeutic applications.
Metabolic Pathways
This compound is involved in several metabolic pathways, including those mediated by cytochrome P450 enzymes. It undergoes hydroxylation and oxidation reactions, leading to the formation of various metabolites . These metabolic transformations can affect the compound’s bioavailability and efficacy, as well as its potential toxicity.
Transport and Distribution
Within cells and tissues, this compound is transported and distributed through interactions with specific transporters and binding proteins. For example, it has been shown to interact with lipid rafts in yeast cells, facilitating its intracellular accumulation . This localization can influence the compound’s activity and function, particularly in targeting specific cellular compartments.
Subcellular Localization
This compound exhibits specific subcellular localization, which can affect its activity and function. In yeast cells, it has been observed to localize to mitochondria, where it induces mitochondrial fission-dependent apoptosis . This targeting is likely mediated by post-translational modifications and specific targeting signals that direct the compound to the mitochondria.
准备方法
Synthetic Routes and Reaction Conditions: The synthesis of piperazine-1-carboxamide can be achieved through various synthetic routes. One common method involves the reaction of piperazine with a suitable carboxylating agent. For example, piperazine can react with phosgene or carbon dioxide in the presence of a base to form this compound. Another method involves the use of carbodiimides as coupling agents to facilitate the formation of the carboxamide bond.
Industrial Production Methods: In industrial settings, the production of this compound often employs continuous flow chemistry techniques. This approach allows for the efficient and scalable synthesis of the compound. For instance, a multi-step flow preparation method can be used, where piperazine is reacted with a carboxylating agent under controlled conditions to yield this compound .
化学反应分析
Types of Reactions: Piperazine-1-carboxamide undergoes various chemical reactions, including:
Oxidation: The compound can be oxidized to form corresponding N-oxides.
Reduction: Reduction reactions can convert this compound to its corresponding amine derivatives.
Substitution: The compound can undergo nucleophilic substitution reactions, where the carboxamide group can be replaced by other functional groups.
Common Reagents and Conditions:
Oxidation: Common oxidizing agents include hydrogen peroxide and peracids.
Reduction: Reducing agents such as lithium aluminum hydride (LiAlH4) or sodium borohydride (NaBH4) are typically used.
Substitution: Nucleophilic reagents such as amines or thiols can be used under basic conditions.
Major Products Formed: The major products formed from these reactions depend on the specific reagents and conditions used. For example, oxidation of this compound can yield N-oxides, while reduction can produce amine derivatives .
科学研究应用
Piperazine-1-carboxamide has a wide range of applications in scientific research:
Chemistry: It serves as a building block for the synthesis of more complex molecules, including pharmaceuticals and agrochemicals.
Biology: The compound is used in the study of enzyme inhibition and protein-ligand interactions.
Medicine: this compound derivatives have been investigated for their potential as antimicrobial, anticancer, and anti-inflammatory agents.
相似化合物的比较
Piperazine: The parent compound, which lacks the carboxamide group.
Piperazine-2-carboxamide: A similar compound with the carboxamide group attached at a different position on the piperazine ring.
N-Substituted Piperazines: Compounds where the nitrogen atoms in the piperazine ring are substituted with various functional groups.
Uniqueness: Piperazine-1-carboxamide is unique due to the presence of the carboxamide group, which imparts specific chemical and biological properties. This functional group enhances the compound’s ability to form hydrogen bonds, increasing its affinity for certain biological targets. Additionally, the carboxamide group can influence the compound’s solubility and stability, making it a valuable scaffold in drug design .
属性
IUPAC Name |
piperazine-1-carboxamide | |
---|---|---|
Source | PubChem | |
URL | https://pubchem.ncbi.nlm.nih.gov | |
Description | Data deposited in or computed by PubChem | |
InChI |
InChI=1S/C5H11N3O/c6-5(9)8-3-1-7-2-4-8/h7H,1-4H2,(H2,6,9) | |
Source | PubChem | |
URL | https://pubchem.ncbi.nlm.nih.gov | |
Description | Data deposited in or computed by PubChem | |
InChI Key |
IVXQBCUBSIPQGU-UHFFFAOYSA-N | |
Source | PubChem | |
URL | https://pubchem.ncbi.nlm.nih.gov | |
Description | Data deposited in or computed by PubChem | |
Canonical SMILES |
C1CN(CCN1)C(=O)N | |
Source | PubChem | |
URL | https://pubchem.ncbi.nlm.nih.gov | |
Description | Data deposited in or computed by PubChem | |
Molecular Formula |
C5H11N3O | |
Source | PubChem | |
URL | https://pubchem.ncbi.nlm.nih.gov | |
Description | Data deposited in or computed by PubChem | |
DSSTOX Substance ID |
DTXSID80293039 | |
Record name | piperazine-1-carboxamide | |
Source | EPA DSSTox | |
URL | https://comptox.epa.gov/dashboard/DTXSID80293039 | |
Description | DSSTox provides a high quality public chemistry resource for supporting improved predictive toxicology. | |
Molecular Weight |
129.16 g/mol | |
Source | PubChem | |
URL | https://pubchem.ncbi.nlm.nih.gov | |
Description | Data deposited in or computed by PubChem | |
CAS No. |
5623-95-0 | |
Record name | 1-Piperazinecarboxamide | |
Source | CAS Common Chemistry | |
URL | https://commonchemistry.cas.org/detail?cas_rn=5623-95-0 | |
Description | CAS Common Chemistry is an open community resource for accessing chemical information. Nearly 500,000 chemical substances from CAS REGISTRY cover areas of community interest, including common and frequently regulated chemicals, and those relevant to high school and undergraduate chemistry classes. This chemical information, curated by our expert scientists, is provided in alignment with our mission as a division of the American Chemical Society. | |
Explanation | The data from CAS Common Chemistry is provided under a CC-BY-NC 4.0 license, unless otherwise stated. | |
Record name | 1-Piperazinecarboxamide | |
Source | ChemIDplus | |
URL | https://pubchem.ncbi.nlm.nih.gov/substance/?source=chemidplus&sourceid=0005623950 | |
Description | ChemIDplus is a free, web search system that provides access to the structure and nomenclature authority files used for the identification of chemical substances cited in National Library of Medicine (NLM) databases, including the TOXNET system. | |
Record name | 1-Piperazinecarboxamide | |
Source | DTP/NCI | |
URL | https://dtp.cancer.gov/dtpstandard/servlet/dwindex?searchtype=NSC&outputformat=html&searchlist=86952 | |
Description | The NCI Development Therapeutics Program (DTP) provides services and resources to the academic and private-sector research communities worldwide to facilitate the discovery and development of new cancer therapeutic agents. | |
Explanation | Unless otherwise indicated, all text within NCI products is free of copyright and may be reused without our permission. Credit the National Cancer Institute as the source. | |
Record name | piperazine-1-carboxamide | |
Source | EPA DSSTox | |
URL | https://comptox.epa.gov/dashboard/DTXSID80293039 | |
Description | DSSTox provides a high quality public chemistry resource for supporting improved predictive toxicology. | |
Record name | 1-PIPERAZINECARBOXAMIDE | |
Source | FDA Global Substance Registration System (GSRS) | |
URL | https://gsrs.ncats.nih.gov/ginas/app/beta/substances/A78BU5W9Y2 | |
Description | The FDA Global Substance Registration System (GSRS) enables the efficient and accurate exchange of information on what substances are in regulated products. Instead of relying on names, which vary across regulatory domains, countries, and regions, the GSRS knowledge base makes it possible for substances to be defined by standardized, scientific descriptions. | |
Explanation | Unless otherwise noted, the contents of the FDA website (www.fda.gov), both text and graphics, are not copyrighted. They are in the public domain and may be republished, reprinted and otherwise used freely by anyone without the need to obtain permission from FDA. Credit to the U.S. Food and Drug Administration as the source is appreciated but not required. | |
Synthesis routes and methods I
Procedure details
Synthesis routes and methods II
Procedure details
Synthesis routes and methods III
Procedure details
Retrosynthesis Analysis
AI-Powered Synthesis Planning: Our tool employs the Template_relevance Pistachio, Template_relevance Bkms_metabolic, Template_relevance Pistachio_ringbreaker, Template_relevance Reaxys, Template_relevance Reaxys_biocatalysis model, leveraging a vast database of chemical reactions to predict feasible synthetic routes.
One-Step Synthesis Focus: Specifically designed for one-step synthesis, it provides concise and direct routes for your target compounds, streamlining the synthesis process.
Accurate Predictions: Utilizing the extensive PISTACHIO, BKMS_METABOLIC, PISTACHIO_RINGBREAKER, REAXYS, REAXYS_BIOCATALYSIS database, our tool offers high-accuracy predictions, reflecting the latest in chemical research and data.
Strategy Settings
Precursor scoring | Relevance Heuristic |
---|---|
Min. plausibility | 0.01 |
Model | Template_relevance |
Template Set | Pistachio/Bkms_metabolic/Pistachio_ringbreaker/Reaxys/Reaxys_biocatalysis |
Top-N result to add to graph | 6 |
Feasible Synthetic Routes
Q1: How do piperazine-1-carboxamide derivatives interact with their targets?
A1: The specific mechanism of action varies depending on the target protein and the substituents on the this compound scaffold. Some derivatives act as antagonists, binding to receptors and blocking the binding of endogenous ligands. For instance, (+)-(2R,5S)-4-[4-cyano-3-(trifluoromethyl)phenyl]-2,5-dimethyl-N-[6-(trifluoromethyl)pyridin-3- yl]this compound (YM580) acts as a potent and peripherally selective androgen receptor (AR) antagonist. [] Others function as enzyme inhibitors, interfering with enzymatic activity. For example, N-(2H-1,3-benzodioxol-5-yl)-4-{thieno[3,2-d]pyrimidin-4-yl}this compound inhibits mitochondrial membrane potential in tumor cells experiencing glucose starvation. []
Q2: What are the downstream effects of these interactions?
A2: Downstream effects are highly dependent on the specific target and its role in cellular pathways. Inhibition of the androgen receptor by YM580 leads to decreased prostate weight in rats, mimicking the effects of surgical castration. [] Blocking TRPM8 channels, as seen with N-(4-tert-butylphenyl)-4-(3-chloropyridin-2-yl)this compound (BCTC), can reduce cold-evoked responses in sensory neurons and modulate inflammatory cytokine expression in bronchial epithelial cells. [, , , ]
Q3: What spectroscopic data are typically used to characterize these compounds?
A4: Common spectroscopic techniques include:* NMR spectroscopy (1H NMR, 13C NMR): Provides information about the structure and connectivity of atoms within the molecule. [, ]* Mass spectrometry (MS): Determines the molecular weight and fragmentation pattern, aiding in structural elucidation. [, ]* Infrared spectroscopy (IR): Identifies functional groups present in the molecule based on their characteristic absorption frequencies. []
Q4: How do modifications to the this compound scaffold affect biological activity?
A5: Substitutions at various positions on the piperazine ring, the nitrogen of the carboxamide group, and the carbonyl group significantly influence potency, selectivity, and pharmacological properties. For example, in a series of thieno[2,3-d]pyrimidine derivatives, N-(4-chloro-3-(trifluoromethyl)phenyl)-4-(6-(4-(4-methylpiperazin-1-yl)phenyl)thieno[2,3-d]pyrimidin-4-yl)this compound (38k) emerged as the most potent VEGFR3 inhibitor. [] This highlights the importance of systematic structural modifications for optimizing desired activities. [, ]
Q5: Are there specific structural features associated with improved potency or selectivity for certain targets?
A6: Yes, SAR studies have revealed key structural features influencing activity. For instance, introducing a bulky substituent at the 4-position of the piperazine ring often enhances activity for certain targets. [, ] Similarly, the presence of electron-withdrawing groups on the aromatic ring attached to the carboxamide nitrogen can increase potency. []
Q6: What is known about the stability of this compound derivatives?
A6: Stability can vary depending on the specific compound and environmental factors like temperature, pH, and exposure to light.
Q7: What formulation strategies are employed to improve stability, solubility, or bioavailability?
A8: Researchers utilize various formulation strategies, including:* Salt formation: Forming hydrochloride salts can improve water solubility and stability. []* Prodrugs: Designing prodrugs that are cleaved in vivo to release the active compound can enhance bioavailability.* Nanoparticle formulations: Encapsulating the compound in nanoparticles can improve solubility, stability, and targeted delivery. []
Q8: What types of in vitro assays are used to assess the biological activity of these compounds?
A9: Various in vitro assays are employed depending on the target, including:* Cell proliferation assays: Measure the ability of the compound to inhibit the growth of cancer cells. [, ] * Reporter gene assays: Assess the compound's ability to modulate the activity of specific signaling pathways. []* **Enzyme inhibition assays: ** Determine the compound's potency in inhibiting the target enzyme. [, ]
Q9: What animal models are used to study the in vivo efficacy of this compound derivatives?
A10: Animal models are selected based on the therapeutic area of interest. For example:* Rodent models of pain: Used to evaluate the analgesic effects of compounds targeting TRPV1 or FAAH. [, ]* Xenograft models of cancer: Assess the ability of compounds to inhibit tumor growth in vivo. []
Q10: What is known about the toxicity and safety profile of this compound derivatives?
A10: Toxicity and safety profiles are compound-specific and require thorough evaluation. Preclinical studies in animals help determine the therapeutic window and potential adverse effects.
Q11: Are there specific structural features associated with increased toxicity?
A11: Certain structural moieties may raise toxicity concerns, such as those known to be metabolically labile or to form reactive metabolites.
Q12: How is computational chemistry used in the development of this compound derivatives?
A13: Computational approaches play a vital role in:* Virtual screening: Identifying potential drug candidates from large databases of chemical structures. []* Molecular docking: Predicting the binding mode and affinity of compounds to their targets. []* Quantitative Structure-Activity Relationship (QSAR) modeling: Developing models to predict the biological activity of novel compounds based on their structural features. [, ]* Molecular dynamics (MD) simulations: Studying the dynamic behavior of compounds and their interactions with target proteins. []
体外研究产品的免责声明和信息
请注意,BenchChem 上展示的所有文章和产品信息仅供信息参考。 BenchChem 上可购买的产品专为体外研究设计,这些研究在生物体外进行。体外研究,源自拉丁语 "in glass",涉及在受控实验室环境中使用细胞或组织进行的实验。重要的是要注意,这些产品没有被归类为药物或药品,他们没有得到 FDA 的批准,用于预防、治疗或治愈任何医疗状况、疾病或疾病。我们必须强调,将这些产品以任何形式引入人类或动物的身体都是法律严格禁止的。遵守这些指南对确保研究和实验的法律和道德标准的符合性至关重要。