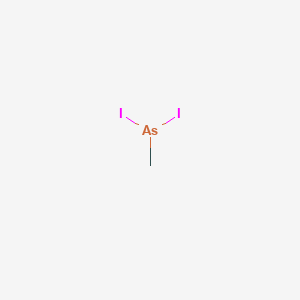
甲基二碘砷
- 点击 快速询问 获取最新报价。
- 提供有竞争力价格的高质量产品,您可以更专注于研究。
描述
Methyldiiodoarsine is identified as an arsenite metabolite . It has a molecular formula of CH3AsI2 and a molecular weight of 343.77 . It is present in both wild type CHO cells and cells lacking excision repair cross-complementing 1 and 2 .
Molecular Structure Analysis
Methyldiiodoarsine contains a total of 6 bonds, including 3 non-H bonds . The 3D chemical structure of Methyldiiodoarsine is based on the ball-and-stick model which displays both the three-dimensional position of the atoms and the bonds between them .科学研究应用
合成二甲基砷酸钠
甲基二碘砷烷在科学研究中的一个重要应用是用于合成二甲基砷酸钠。在Jagadish, Aposhian, & Mash (2003)的一项研究中,甲基二碘砷烷被甲基化为碘甲烷-14C,从而生成二甲基砷酸钠。这种高效的合成突显了甲基二碘砷烷在生产各种研究应用衍生物中的作用。
在神经疾病和癫痫治疗中的作用
在另一个背景下,甲基二碘砷烷的衍生物已被研究与神经疾病,特别是癫痫治疗相关。Citraro et al. (2014)的一篇论文讨论了激活某些受体作为抗癫痫药物的靶点,间接涉及更广泛的有机砷化合物领域,包括甲基二碘砷烷衍生物。
甲基化药代遗传学
甲基二碘砷烷在甲基化药代遗传学研究中也很重要。Weinshilboum、Otterness和Szumlanski(1999)在他们关于methylation pharmacogenetics的研究中探讨了甲基化在药物代谢中的作用以及基因变异的影响。这一研究领域涵盖了像甲基二碘砷烷这样的化合物及其代谢影响的研究。
砷代谢及其影响
一项由Sun, Liu, & Cai (2016)进行的研究探讨了砷代谢,突出了甲基化砷类化合物的作用,其中可能包括甲基二碘砷烷衍生物。这项研究对于理解砷的毒理学和治疗效应至关重要,而甲基二碘砷烷衍生物可能发挥作用。
癌症中的甲基化研究
蛋白质甲基化领域,在其中可能包括对甲基二碘砷烷衍生物的研究,在癌症研究中具有重要意义。Paik, Paik, & Kim (2007)和Murn & Shi (2017)的论文讨论了蛋白质甲基化研究的里程碑和新领域,强调了其在细胞功能中的重要性以及在癌症治疗中的潜力。
安全和危害
属性
IUPAC Name |
diiodo(methyl)arsane |
Source
|
---|---|---|
Source | PubChem | |
URL | https://pubchem.ncbi.nlm.nih.gov | |
Description | Data deposited in or computed by PubChem | |
InChI |
InChI=1S/CH3AsI2/c1-2(3)4/h1H3 |
Source
|
Source | PubChem | |
URL | https://pubchem.ncbi.nlm.nih.gov | |
Description | Data deposited in or computed by PubChem | |
InChI Key |
ZKGSUVMABCXPKK-UHFFFAOYSA-N |
Source
|
Source | PubChem | |
URL | https://pubchem.ncbi.nlm.nih.gov | |
Description | Data deposited in or computed by PubChem | |
Canonical SMILES |
C[As](I)I |
Source
|
Source | PubChem | |
URL | https://pubchem.ncbi.nlm.nih.gov | |
Description | Data deposited in or computed by PubChem | |
Molecular Formula |
CH3AsI2 |
Source
|
Source | PubChem | |
URL | https://pubchem.ncbi.nlm.nih.gov | |
Description | Data deposited in or computed by PubChem | |
DSSTOX Substance ID |
DTXSID40992798 |
Source
|
Record name | Methylarsonous diiodide | |
Source | EPA DSSTox | |
URL | https://comptox.epa.gov/dashboard/DTXSID40992798 | |
Description | DSSTox provides a high quality public chemistry resource for supporting improved predictive toxicology. | |
Molecular Weight |
343.765 g/mol |
Source
|
Source | PubChem | |
URL | https://pubchem.ncbi.nlm.nih.gov | |
Description | Data deposited in or computed by PubChem | |
Product Name |
Methyldiiodoarsine | |
CAS RN |
7207-97-8 |
Source
|
Record name | Arsonous diiodide, methyl- | |
Source | CAS Common Chemistry | |
URL | https://commonchemistry.cas.org/detail?cas_rn=7207-97-8 | |
Description | CAS Common Chemistry is an open community resource for accessing chemical information. Nearly 500,000 chemical substances from CAS REGISTRY cover areas of community interest, including common and frequently regulated chemicals, and those relevant to high school and undergraduate chemistry classes. This chemical information, curated by our expert scientists, is provided in alignment with our mission as a division of the American Chemical Society. | |
Explanation | The data from CAS Common Chemistry is provided under a CC-BY-NC 4.0 license, unless otherwise stated. | |
Record name | Arsine, diiodomethyl- | |
Source | ChemIDplus | |
URL | https://pubchem.ncbi.nlm.nih.gov/substance/?source=chemidplus&sourceid=0007207978 | |
Description | ChemIDplus is a free, web search system that provides access to the structure and nomenclature authority files used for the identification of chemical substances cited in National Library of Medicine (NLM) databases, including the TOXNET system. | |
Record name | Methylarsonous diiodide | |
Source | EPA DSSTox | |
URL | https://comptox.epa.gov/dashboard/DTXSID40992798 | |
Description | DSSTox provides a high quality public chemistry resource for supporting improved predictive toxicology. | |
体外研究产品的免责声明和信息
请注意,BenchChem 上展示的所有文章和产品信息仅供信息参考。 BenchChem 上可购买的产品专为体外研究设计,这些研究在生物体外进行。体外研究,源自拉丁语 "in glass",涉及在受控实验室环境中使用细胞或组织进行的实验。重要的是要注意,这些产品没有被归类为药物或药品,他们没有得到 FDA 的批准,用于预防、治疗或治愈任何医疗状况、疾病或疾病。我们必须强调,将这些产品以任何形式引入人类或动物的身体都是法律严格禁止的。遵守这些指南对确保研究和实验的法律和道德标准的符合性至关重要。