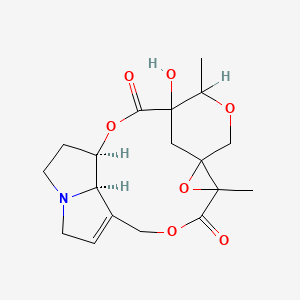
Adonifoline
描述
Adonifoline is a rare natural compound that belongs to the class of carbamate alkaloids. . This compound has garnered attention due to its potential pharmacological activities, particularly in the field of cardiovascular health.
作用机制
Target of Action
Adonifoline, a pyrrolizidine alkaloid (PA), primarily targets the liver . PAs are known to be hepatotoxic, meaning they can cause damage to the liver . The liver is a vital organ that plays a key role in metabolism, detoxification, and other essential physiological processes.
Mode of Action
This compound interacts with its targets by undergoing metabolic activation in the liver . This process leads to the formation of toxic metabolites, which can bind to DNA or proteins, causing cellular damage
Pharmacokinetics
The pharmacokinetics of this compound involves its absorption, distribution, metabolism, and excretion (ADME). Upon administration, this compound is quickly absorbed and metabolized to form various metabolites . It has been observed that this compound has a lower bioavailability compared to other PAs
Result of Action
The primary result of this compound’s action is hepatotoxicity, or liver damage . This is due to the formation of toxic metabolites during the metabolism of this compound, which can bind to DNA or proteins, leading to cellular damage . The extent of this damage can vary depending on the dose and duration of exposure.
Action Environment
Environmental factors can influence the action, efficacy, and stability of this compound. Factors such as the route of administration, the presence of other substances, and individual physiological differences can all impact how this compound is metabolized and its subsequent effects . .
生化分析
Biochemical Properties
Adonifoline plays a significant role in biochemical reactions, particularly in the liver. It interacts with various enzymes, proteins, and other biomolecules. One of the primary interactions of this compound is with cytochrome P450 enzymes, which are responsible for its metabolic activation. The compound undergoes bioactivation to form reactive metabolites that can bind to cellular macromolecules, leading to hepatotoxicity . Additionally, this compound interacts with glutathione, a critical antioxidant, leading to the formation of glutathione conjugates . These interactions highlight the compound’s potential to cause oxidative stress and cellular damage.
Cellular Effects
This compound exerts various effects on different types of cells and cellular processes. In hepatocytes, this compound induces cytotoxicity by disrupting cellular metabolism and causing oxidative stress . The compound affects cell signaling pathways, including the activation of stress-activated protein kinases and the inhibition of cell survival pathways . This compound also influences gene expression by upregulating genes involved in oxidative stress response and downregulating genes associated with cell survival . These cellular effects contribute to the compound’s hepatotoxic properties.
Molecular Mechanism
The molecular mechanism of this compound involves its bioactivation by cytochrome P450 enzymes to form reactive metabolites . These metabolites can covalently bind to cellular proteins, DNA, and other macromolecules, leading to cellular damage and apoptosis . This compound also inhibits the activity of key enzymes involved in cellular detoxification, such as glutathione S-transferase . This inhibition further exacerbates oxidative stress and cellular damage. Additionally, this compound can induce mitochondrial dysfunction, leading to the release of pro-apoptotic factors and the activation of apoptotic pathways .
Temporal Effects in Laboratory Settings
In laboratory settings, the effects of this compound change over time. The compound’s stability and degradation play a crucial role in its long-term effects on cellular function. This compound is relatively stable under physiological conditions, but its reactive metabolites can accumulate over time, leading to sustained cellular damage . Long-term exposure to this compound in in vitro and in vivo studies has shown persistent oxidative stress, mitochondrial dysfunction, and apoptosis . These temporal effects highlight the importance of monitoring this compound’s stability and degradation in laboratory settings.
Dosage Effects in Animal Models
The effects of this compound vary with different dosages in animal models. At low doses, this compound induces mild oxidative stress and cellular damage . At higher doses, the compound’s hepatotoxic effects become more pronounced, leading to severe liver damage and apoptosis . Threshold effects have been observed, where a specific dosage level triggers a significant increase in toxicity. Additionally, high doses of this compound can cause systemic toxicity, affecting other organs and tissues . These dosage effects underscore the importance of determining safe and effective dosage levels for potential therapeutic applications.
Metabolic Pathways
This compound is involved in various metabolic pathways, primarily in the liver. The compound undergoes phase I and phase II metabolic processes, including oxidation, reduction, and conjugation reactions . Cytochrome P450 enzymes play a crucial role in the bioactivation of this compound, leading to the formation of reactive metabolites . These metabolites can undergo further conjugation with glutathione, glucuronic acid, or sulfate, facilitating their excretion from the body . The metabolic pathways of this compound highlight its potential to cause oxidative stress and cellular damage through the formation of reactive intermediates.
Transport and Distribution
This compound is transported and distributed within cells and tissues through various mechanisms. The compound can enter cells via passive diffusion or active transport mediated by specific transporters . Once inside the cells, this compound can bind to cellular proteins and macromolecules, affecting its localization and accumulation . The compound’s distribution within tissues is influenced by its lipophilicity and binding affinity to cellular components . These transport and distribution mechanisms play a crucial role in determining the compound’s cellular effects and toxicity.
Subcellular Localization
The subcellular localization of this compound is critical for its activity and function. The compound can localize to various cellular compartments, including the cytoplasm, mitochondria, and nucleus . This compound’s localization to the mitochondria is particularly significant, as it can induce mitochondrial dysfunction and oxidative stress . Additionally, the compound’s interaction with nuclear proteins can affect gene expression and cellular signaling pathways . The subcellular localization of this compound highlights its potential to cause cellular damage through multiple mechanisms.
准备方法
Adonifoline can be extracted from the Adonis vernalis plant. The general extraction method involves solvent extraction, followed by separation and purification The specific preparation method may vary depending on the researcher and experimental conditions
化学反应分析
Adonifoline undergoes various chemical reactions, including oxidation, reduction, and substitution. Common reagents and conditions used in these reactions include strong oxidizing agents for oxidation reactions and reducing agents for reduction reactions. The major products formed from these reactions depend on the specific conditions and reagents used. Detailed studies on the specific reaction pathways and products of this compound are limited, necessitating further research.
科学研究应用
Adonifoline has shown potential in several scientific research applications:
Chemistry: this compound is studied for its unique chemical structure and reactivity, contributing to the understanding of carbamate alkaloids.
Biology: Research on this compound includes its effects on biological systems, particularly its interaction with cellular components.
相似化合物的比较
Adonifoline is compared with other pyrrolizidine alkaloids, such as senecionine and retrorsine. These compounds share similar chemical structures but differ in their pharmacokinetic behavior and toxicity. For instance, senecionine has been found to have more potent toxicity compared to this compound . The unique properties of this compound, such as its specific pharmacological activities and lower toxicity, distinguish it from other similar compounds.
Conclusion
This compound is a rare and intriguing compound with potential applications in various scientific fields. Its unique chemical structure and pharmacological activities make it a subject of interest for further research. Understanding its preparation methods, chemical reactions, and mechanism of action will contribute to the development of new therapeutic strategies and industrial applications.
属性
IUPAC Name |
(1R,20R)-4-hydroxy-5,10-dimethyl-2,6,9,12-tetraoxa-17-azapentacyclo[12.5.1.14,8.08,10.017,20]henicos-14-ene-3,11-dione | |
---|---|---|
Source | PubChem | |
URL | https://pubchem.ncbi.nlm.nih.gov | |
Description | Data deposited in or computed by PubChem | |
InChI |
InChI=1S/C18H23NO7/c1-10-18(22)8-17(9-24-10)16(2,26-17)14(20)23-7-11-3-5-19-6-4-12(13(11)19)25-15(18)21/h3,10,12-13,22H,4-9H2,1-2H3/t10?,12-,13-,16?,17?,18?/m1/s1 | |
Source | PubChem | |
URL | https://pubchem.ncbi.nlm.nih.gov | |
Description | Data deposited in or computed by PubChem | |
InChI Key |
MYOFCWPLRKBPJD-AQYQBICXSA-N | |
Source | PubChem | |
URL | https://pubchem.ncbi.nlm.nih.gov | |
Description | Data deposited in or computed by PubChem | |
Canonical SMILES |
CC1C2(CC3(CO1)C(O3)(C(=O)OCC4=CCN5C4C(CC5)OC2=O)C)O | |
Source | PubChem | |
URL | https://pubchem.ncbi.nlm.nih.gov | |
Description | Data deposited in or computed by PubChem | |
Isomeric SMILES |
CC1C2(CC3(CO1)C(O3)(C(=O)OCC4=CCN5[C@H]4[C@@H](CC5)OC2=O)C)O | |
Source | PubChem | |
URL | https://pubchem.ncbi.nlm.nih.gov | |
Description | Data deposited in or computed by PubChem | |
Molecular Formula |
C18H23NO7 | |
Source | PubChem | |
URL | https://pubchem.ncbi.nlm.nih.gov | |
Description | Data deposited in or computed by PubChem | |
Molecular Weight |
365.4 g/mol | |
Source | PubChem | |
URL | https://pubchem.ncbi.nlm.nih.gov | |
Description | Data deposited in or computed by PubChem | |
Retrosynthesis Analysis
AI-Powered Synthesis Planning: Our tool employs the Template_relevance Pistachio, Template_relevance Bkms_metabolic, Template_relevance Pistachio_ringbreaker, Template_relevance Reaxys, Template_relevance Reaxys_biocatalysis model, leveraging a vast database of chemical reactions to predict feasible synthetic routes.
One-Step Synthesis Focus: Specifically designed for one-step synthesis, it provides concise and direct routes for your target compounds, streamlining the synthesis process.
Accurate Predictions: Utilizing the extensive PISTACHIO, BKMS_METABOLIC, PISTACHIO_RINGBREAKER, REAXYS, REAXYS_BIOCATALYSIS database, our tool offers high-accuracy predictions, reflecting the latest in chemical research and data.
Strategy Settings
Precursor scoring | Relevance Heuristic |
---|---|
Min. plausibility | 0.01 |
Model | Template_relevance |
Template Set | Pistachio/Bkms_metabolic/Pistachio_ringbreaker/Reaxys/Reaxys_biocatalysis |
Top-N result to add to graph | 6 |
Feasible Synthetic Routes
体外研究产品的免责声明和信息
请注意,BenchChem 上展示的所有文章和产品信息仅供信息参考。 BenchChem 上可购买的产品专为体外研究设计,这些研究在生物体外进行。体外研究,源自拉丁语 "in glass",涉及在受控实验室环境中使用细胞或组织进行的实验。重要的是要注意,这些产品没有被归类为药物或药品,他们没有得到 FDA 的批准,用于预防、治疗或治愈任何医疗状况、疾病或疾病。我们必须强调,将这些产品以任何形式引入人类或动物的身体都是法律严格禁止的。遵守这些指南对确保研究和实验的法律和道德标准的符合性至关重要。