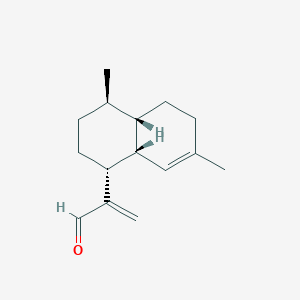
青蒿醛
描述
Artemisinic aldehyde is a sesquiterpene aldehyde found in the plant Artemisia annua, also known as sweet wormwood. The plant is native to Asia, but is now widely cultivated in many parts of the world. Artemisinic aldehyde has been studied extensively for its medicinal properties, and has been found to be a promising candidate for the treatment of malaria and other diseases.
科学研究应用
青蒿素生物合成中的青蒿醛
青蒿素是从植物青蒿中提取的一种对治疟疾至关重要的化合物。了解其生物合成对于增加其产量至关重要。青蒿醛在这一生物合成途径中作为前体发挥着重要作用。将青蒿醛转化为相应的酸是青蒿素合成中至关重要的过程,涉及到特定的酶,如在青蒿的毛状结构中高度表达的醛脱氢酶同源物。
在其他植物中工程化青蒿素前体
研究已经探索了在转基因植物(如烟草)中生产青蒿素前体(如青蒿醛)的方法。这种方法涉及在烟草中表达来自青蒿的特定基因,导致关键中间体如青蒿醇和二氢青蒿醇的积累。这些研究突显了通过代谢工程利用替代植物宿主生产青蒿素前体的潜力。
安全和危害
未来方向
作用机制
Artemisinic aldehyde, also known as artemisic aldehyde, is a key intermediate in the biosynthesis of artemisinin, a potent anti-malarial compound . This article will cover the mechanism of action of artemisinic aldehyde, including its targets, mode of action, biochemical pathways, pharmacokinetics, results of action, and the influence of environmental factors.
Target of Action
Artemisinic aldehyde primarily targets the enzymes DBR2 (artemisinic aldehyde Δ11 (13) reductase) and ALDH1 (aldehyde dehydrogenase 1) . These enzymes play a crucial role in the biosynthesis of artemisinin, a compound highly effective against the malaria parasite Plasmodium falciparum .
Mode of Action
Artemisinic aldehyde interacts with its targets, DBR2 and ALDH1, to generate dihydroartemisinic aldehyde (DHAAA) and dihydroartemisinic acid (DHAA), respectively . This interaction results in the conversion of artemisinic aldehyde into DHAAA and subsequently DHAA, which are key steps in the biosynthesis of artemisinin .
Biochemical Pathways
The biochemical pathway of artemisinin biosynthesis, in which artemisinic aldehyde plays a key role, has been completely elucidated . Artemisinic aldehyde is catalyzed by DBR2 to generate DHAAA, and DHAAA is then converted to DHAA by ALDH1 . This pathway is crucial for the production of artemisinin, a potent anti-malarial compound .
Pharmacokinetics
Information on the ADME properties (Absorption, Distribution, Metabolism, and Excretion) of artemisinic aldehyde is currently limited. It is known that artemisinic aldehyde is a lipophilic compound, which may influence its absorption and distribution .
Result of Action
The result of the action of artemisinic aldehyde is the production of DHAAA and subsequently DHAA . These compounds are precursors in the biosynthesis of artemisinin, which has potent anti-malarial activity . Additionally, artemisinin and its derivatives have demonstrated anticancer, anti-inflammation, antiviral, and anti-SARS-CoV-2 activity .
Action Environment
The action of artemisinic aldehyde can be influenced by various environmental factors. For instance, the biosynthesis of artemisinin, in which artemisinic aldehyde plays a key role, occurs in the glandular trichomes of the plant Artemisia annua . Therefore, factors that affect the growth and development of these trichomes could potentially influence the action of artemisinic aldehyde.
生化分析
Biochemical Properties
Artemisinic aldehyde plays a significant role in the biosynthesis of artemisinin. It interacts with several enzymes and proteins during this process. One of the key enzymes involved is artemisinic aldehyde Δ11(13) reductase (DBR2), which reduces artemisinic aldehyde to dihydroartemisinic aldehyde . Another important enzyme is cytochrome P450 monooxygenase (CYP71AV1), which oxidizes artemisinic aldehyde to artemisinic acid . These interactions are crucial for the conversion of artemisinic aldehyde into its downstream products, which eventually lead to the formation of artemisinin.
Cellular Effects
Artemisinic aldehyde has been shown to influence various cellular processes. It affects cell signaling pathways, gene expression, and cellular metabolism. For instance, artemisinic aldehyde can modulate the expression of genes involved in the biosynthesis of artemisinin, thereby affecting the overall production of this compound . Additionally, artemisinic aldehyde has been reported to impact cellular metabolism by interacting with enzymes involved in metabolic pathways, leading to changes in metabolite levels and metabolic flux .
Molecular Mechanism
The molecular mechanism of artemisinic aldehyde involves its interactions with specific enzymes and proteins. Artemisinic aldehyde binds to the active site of DBR2, where it undergoes reduction to form dihydroartemisinic aldehyde . This reaction is facilitated by the presence of cofactors such as NADPH. Similarly, artemisinic aldehyde is oxidized by CYP71AV1 to form artemisinic acid, a precursor of artemisinin . These binding interactions and enzymatic reactions are essential for the conversion of artemisinic aldehyde into its downstream products.
Temporal Effects in Laboratory Settings
In laboratory settings, the effects of artemisinic aldehyde can change over time. The stability and degradation of artemisinic aldehyde are important factors that influence its long-term effects on cellular function. Studies have shown that artemisinic aldehyde can be stable under certain conditions, but it may degrade over time, leading to a decrease in its concentration and effectiveness . Long-term exposure to artemisinic aldehyde in in vitro and in vivo studies has also been reported to affect cellular function, including changes in gene expression and metabolic activity .
Dosage Effects in Animal Models
The effects of artemisinic aldehyde can vary with different dosages in animal models. At low doses, artemisinic aldehyde has been shown to have beneficial effects, such as enhancing the production of artemisinin . At high doses, artemisinic aldehyde may exhibit toxic or adverse effects, including cytotoxicity and disruption of cellular processes . Threshold effects have also been observed, where the impact of artemisinic aldehyde on cellular function changes significantly at certain dosage levels .
Metabolic Pathways
Artemisinic aldehyde is involved in several metabolic pathways, including the biosynthesis of artemisinin. It interacts with enzymes such as DBR2 and CYP71AV1, which catalyze its conversion into dihydroartemisinic aldehyde and artemisinic acid, respectively . These metabolic pathways are essential for the production of artemisinin and its derivatives. Additionally, artemisinic aldehyde can affect metabolic flux and metabolite levels by modulating the activity of enzymes involved in these pathways .
Transport and Distribution
The transport and distribution of artemisinic aldehyde within cells and tissues are critical for its activity and function. Artemisinic aldehyde is transported across cellular membranes by specific transporters and binding proteins . These transporters facilitate the movement of artemisinic aldehyde to different cellular compartments, where it can interact with enzymes and other biomolecules. The localization and accumulation of artemisinic aldehyde within cells can also influence its effectiveness and stability .
Subcellular Localization
Artemisinic aldehyde is localized in specific subcellular compartments, which can affect its activity and function. It is primarily found in the glandular trichomes of Artemisia annua, where it undergoes biosynthesis and conversion into artemisinin . The subcellular localization of artemisinic aldehyde is regulated by targeting signals and post-translational modifications that direct it to specific compartments or organelles . These localization mechanisms are essential for the proper functioning of artemisinic aldehyde in biochemical reactions and metabolic pathways.
属性
IUPAC Name |
2-[(1R,4R,4aS,8aR)-4,7-dimethyl-1,2,3,4,4a,5,6,8a-octahydronaphthalen-1-yl]prop-2-enal | |
---|---|---|
Source | PubChem | |
URL | https://pubchem.ncbi.nlm.nih.gov | |
Description | Data deposited in or computed by PubChem | |
InChI |
InChI=1S/C15H22O/c1-10-4-6-13-11(2)5-7-14(12(3)9-16)15(13)8-10/h8-9,11,13-15H,3-7H2,1-2H3/t11-,13+,14+,15+/m1/s1 | |
Source | PubChem | |
URL | https://pubchem.ncbi.nlm.nih.gov | |
Description | Data deposited in or computed by PubChem | |
InChI Key |
SVAPNGMAOHQQFJ-UNQGMJICSA-N | |
Source | PubChem | |
URL | https://pubchem.ncbi.nlm.nih.gov | |
Description | Data deposited in or computed by PubChem | |
Canonical SMILES |
CC1CCC(C2C1CCC(=C2)C)C(=C)C=O | |
Source | PubChem | |
URL | https://pubchem.ncbi.nlm.nih.gov | |
Description | Data deposited in or computed by PubChem | |
Isomeric SMILES |
C[C@@H]1CC[C@H]([C@@H]2[C@H]1CCC(=C2)C)C(=C)C=O | |
Source | PubChem | |
URL | https://pubchem.ncbi.nlm.nih.gov | |
Description | Data deposited in or computed by PubChem | |
Molecular Formula |
C15H22O | |
Source | PubChem | |
URL | https://pubchem.ncbi.nlm.nih.gov | |
Description | Data deposited in or computed by PubChem | |
Molecular Weight |
218.33 g/mol | |
Source | PubChem | |
URL | https://pubchem.ncbi.nlm.nih.gov | |
Description | Data deposited in or computed by PubChem | |
Retrosynthesis Analysis
AI-Powered Synthesis Planning: Our tool employs the Template_relevance Pistachio, Template_relevance Bkms_metabolic, Template_relevance Pistachio_ringbreaker, Template_relevance Reaxys, Template_relevance Reaxys_biocatalysis model, leveraging a vast database of chemical reactions to predict feasible synthetic routes.
One-Step Synthesis Focus: Specifically designed for one-step synthesis, it provides concise and direct routes for your target compounds, streamlining the synthesis process.
Accurate Predictions: Utilizing the extensive PISTACHIO, BKMS_METABOLIC, PISTACHIO_RINGBREAKER, REAXYS, REAXYS_BIOCATALYSIS database, our tool offers high-accuracy predictions, reflecting the latest in chemical research and data.
Strategy Settings
Precursor scoring | Relevance Heuristic |
---|---|
Min. plausibility | 0.01 |
Model | Template_relevance |
Template Set | Pistachio/Bkms_metabolic/Pistachio_ringbreaker/Reaxys/Reaxys_biocatalysis |
Top-N result to add to graph | 6 |
Feasible Synthetic Routes
Q & A
Q1: What is the role of artemisinic aldehyde in artemisinin biosynthesis?
A1: Artemisinic aldehyde is a crucial branch point in the artemisinin biosynthetic pathway [, , ]. It can be converted to artemisinic acid by amorphadiene oxidase (AMO), leading to the production of arteannuin B. Alternatively, artemisinic aldehyde Δ11(13) reductase (DBR2) can reduce it to dihydroartemisinic aldehyde, which is further oxidized to dihydroartemisinic acid (DHAA), the precursor of artemisinin [, ].
Q2: Can the production of artemisinin be enhanced by manipulating the enzymes involved in artemisinic aldehyde metabolism?
A2: Yes, research suggests that manipulating the expression of enzymes like DBR2 can influence artemisinin yield. Overexpressing DBR2 in Artemisia annua has been shown to increase the levels of both artemisinin and its precursor DHAA [, , ]. Inhibiting branching pathways that compete with artemisinin biosynthesis, such as those leading to β-caryophyllene or β-farnesene, can also enhance artemisinin production [].
Q3: Is the activity of DBR2 influenced by factors beyond its expression level?
A3: Recent studies have revealed that variations in the promoter region of the DBR2 gene can significantly affect its expression level and, consequently, artemisinin yield in different chemotypes of Artemisia annua [, , ]. This finding highlights the importance of considering genetic factors beyond gene expression when engineering artemisinin biosynthesis.
Q4: Can artemisinin or its precursors be produced in organisms other than Artemisia annua?
A4: Research shows promising results for heterologous production. For instance, expressing the artemisinin pathway genes in yeast (Saccharomyces cerevisiae) has enabled the production of DHAA [, ]. Similarly, introducing these genes into tobacco plants resulted in the accumulation of artemisinin precursors, including artemisinic alcohol and dihydroartemisinic alcohol [, ].
Q5: What challenges remain in engineering artemisinin biosynthesis in heterologous hosts?
A5: While promising, heterologous production faces hurdles. In tobacco, for example, the pathway seems to favor the reduction of aldehydes to alcohols rather than their oxidation to acids, limiting DHAA accumulation []. Additionally, glycosylation and glutathione conjugation of pathway intermediates can divert metabolic flux away from DHAA [].
Q6: What is the function of artemisinic aldehyde Δ11(13) reductase (DBR2)?
A6: DBR2, found in the glandular trichomes of Artemisia annua, catalyzes the reduction of the Δ11(13) double bond in artemisinic aldehyde, yielding dihydroartemisinic aldehyde [, ]. This reduction is a critical step in directing the pathway towards artemisinin production.
Q7: Are there DBR2 homologs in other Artemisia species?
A7: While other Artemisia species don't produce artemisinin, research has identified a DBR2 homolog (abDBR2) in Artemisia absinthium [, ]. This homolog exhibits comparable activity to A. annua DBR2 in converting artemisinic aldehyde to dihydroartemisinic aldehyde. Notably, dihydroartemisinic aldehyde was detected in A. absinthium leaves fed with artemisinic aldehyde, suggesting active abDBR2 in planta [].
Q8: What are the implications of finding functional DBR2 homologs in non-artemisinin producing plants?
A8: The presence of functional DBR2 homologs in species like A. absinthium suggests these plants might possess a partial artemisinin-producing ability [, ]. This discovery opens avenues for exploring the use of metabolic engineering to induce artemisinin production in these alternative hosts.
Q9: What are the phytochemical differences between high- and low-artemisinin producing (HAP and LAP) chemotypes of Artemisia annua?
A10: Analysis of HAP and LAP chemotypes revealed distinct terpenoid profiles []. The LAP chemotype, characterized by low DBR2 expression, accumulates higher levels of artemisinic acid, arteannuin B and other sesquiterpenes unsaturated at the 11,13-position []. In contrast, the HAP chemotype, with higher DBR2 expression, shows higher levels of DHAA, artemisinin, and other sesquiterpenes saturated at the 11,13-position []. This difference highlights the role of DBR2 in controlling the metabolic flux towards artemisinin biosynthesis.
Q10: What are some future research directions in the study of artemisinic aldehyde?
A10: Future research could focus on:
- Exploring transport and sequestration: Understanding the mechanisms involved in transporting and sequestering artemisinic aldehyde and its derivatives within the cell and to the apoplast [].
- Investigating the role of transcription factors: Identifying and manipulating transcription factors that regulate DBR2 and other genes in the artemisinin pathway [, ].
体外研究产品的免责声明和信息
请注意,BenchChem 上展示的所有文章和产品信息仅供信息参考。 BenchChem 上可购买的产品专为体外研究设计,这些研究在生物体外进行。体外研究,源自拉丁语 "in glass",涉及在受控实验室环境中使用细胞或组织进行的实验。重要的是要注意,这些产品没有被归类为药物或药品,他们没有得到 FDA 的批准,用于预防、治疗或治愈任何医疗状况、疾病或疾病。我们必须强调,将这些产品以任何形式引入人类或动物的身体都是法律严格禁止的。遵守这些指南对确保研究和实验的法律和道德标准的符合性至关重要。