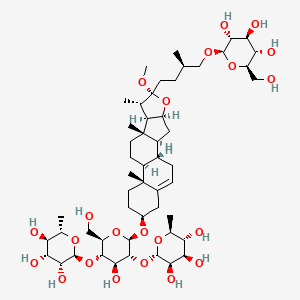
原薯蓣皂苷元
描述
原薯蓣皂苷元甲酯是一种天然化合物,存在于薯蓣科植物的根茎中,薯蓣科是一类山药植物。 它是一种薯蓣皂苷,以其生物活性而闻名,包括抗炎和抗癌活性 . 该化合物已被研究其潜在的治疗效果,特别是在治疗各种炎症和癌症方面 .
科学研究应用
原薯蓣皂苷元甲酯具有广泛的科学研究应用,包括:
作用机制
原薯蓣皂苷元甲酯通过多个分子靶点和途径发挥作用。 在癌细胞中,它调节丝裂原活化蛋白激酶 (MAPK) 信号通路,导致细胞凋亡的诱导和细胞增殖的抑制 . 它还破坏脂筏并降低胆固醇浓度,这进一步抑制癌细胞生长 . 在炎症性疾病中,原薯蓣皂苷元甲酯降低核因子κB (NF-κB) 的活化和促炎细胞因子的表达,从而减轻炎症 .
生化分析
Biochemical Properties
Methylprotodioscin plays a crucial role in various biochemical reactions. It interacts with several enzymes, proteins, and other biomolecules, influencing their activity and function. One of the primary interactions of Methylprotodioscin is with the enzyme superoxide dismutase 2, which is involved in the detoxification of reactive oxygen species. Methylprotodioscin has been shown to elevate the levels of superoxide dismutase 2, thereby enhancing the cell’s antioxidant defense mechanism . Additionally, Methylprotodioscin inhibits the enzyme mitochondrial E3 ubiquitin ligase 1, which is involved in the regulation of mitochondrial function and apoptosis . These interactions highlight the compound’s role in modulating oxidative stress and apoptosis pathways.
Cellular Effects
Methylprotodioscin exerts significant effects on various types of cells and cellular processes. In cancer cells, such as pancreatic cancer cells and human lung cancer cells, Methylprotodioscin has been shown to inhibit cell proliferation and induce apoptosis . This compound influences cell signaling pathways, including the p38 mitogen-activated protein kinase pathway, which is involved in the regulation of cell growth and apoptosis . Methylprotodioscin also affects gene expression by upregulating apoptotic-related proteins such as cleaved caspase-3 and cleaved caspase-9, and downregulating anti-apoptotic proteins like Bcl-2 . Furthermore, Methylprotodioscin impacts cellular metabolism by suppressing glycolysis, thereby reducing the energy supply to cancer cells and promoting cell death .
Molecular Mechanism
The molecular mechanism of Methylprotodioscin involves several key interactions at the molecular level. Methylprotodioscin binds to specific biomolecules, such as the proteins NIX and LC3, which are involved in the process of mitophagy . This binding interaction leads to the upregulation of mitophagy-related proteins and the induction of mitochondrial dysfunction, ultimately resulting in apoptosis . Additionally, Methylprotodioscin inhibits the activity of the enzyme p38 mitogen-activated protein kinase, which plays a critical role in cell survival and apoptosis . By modulating these molecular pathways, Methylprotodioscin exerts its anticancer effects and promotes cell death in cancer cells.
Temporal Effects in Laboratory Settings
In laboratory settings, the effects of Methylprotodioscin have been observed to change over time. Studies have shown that Methylprotodioscin is relatively stable under standard laboratory conditions, with minimal degradation over time . The long-term effects of Methylprotodioscin on cellular function have been observed in both in vitro and in vivo studies. In in vitro studies, prolonged exposure to Methylprotodioscin has been shown to result in sustained inhibition of cell proliferation and induction of apoptosis . In in vivo studies, Methylprotodioscin has demonstrated long-term anticancer effects, with significant tumor growth inhibition observed over extended periods .
Dosage Effects in Animal Models
The effects of Methylprotodioscin vary with different dosages in animal models. Studies have shown that low to moderate doses of Methylprotodioscin exhibit significant anticancer effects, with minimal toxicity . At high doses, Methylprotodioscin can induce toxic effects, including hepatotoxicity and nephrotoxicity . Threshold effects have also been observed, where a certain dosage level is required to achieve the desired therapeutic effect. These findings highlight the importance of optimizing the dosage of Methylprotodioscin to maximize its therapeutic benefits while minimizing potential adverse effects.
Metabolic Pathways
Methylprotodioscin is involved in several metabolic pathways, interacting with various enzymes and cofactors. One of the primary metabolic pathways of Methylprotodioscin involves its conversion to protodioscin, a related saponin with similar pharmacological properties . This conversion is mediated by specific enzymes, including glycosidases, which cleave the glycosidic bonds in Methylprotodioscin . Additionally, Methylprotodioscin affects metabolic flux by modulating the activity of key metabolic enzymes, such as those involved in glycolysis and oxidative phosphorylation . These interactions influence the overall metabolic profile of cells and contribute to the compound’s therapeutic effects.
Transport and Distribution
The transport and distribution of Methylprotodioscin within cells and tissues are mediated by various transporters and binding proteins. Methylprotodioscin is known to interact with specific transporters, such as the organic anion-transporting polypeptides, which facilitate its uptake into cells . Once inside the cells, Methylprotodioscin can bind to intracellular proteins, influencing its localization and accumulation . The distribution of Methylprotodioscin within tissues is also influenced by factors such as blood flow and tissue permeability . These factors determine the overall bioavailability and therapeutic efficacy of Methylprotodioscin.
Subcellular Localization
The subcellular localization of Methylprotodioscin plays a crucial role in its activity and function. Methylprotodioscin has been shown to localize primarily to the mitochondria, where it exerts its effects on mitochondrial function and apoptosis . The targeting of Methylprotodioscin to the mitochondria is mediated by specific targeting signals and post-translational modifications . Additionally, Methylprotodioscin can localize to other subcellular compartments, such as the cytosol and nucleus, where it influences various cellular processes . The subcellular localization of Methylprotodioscin is essential for its therapeutic effects and determines its overall efficacy in different cellular contexts.
准备方法
合成路线和反应条件
原薯蓣皂苷元甲酯的合成涉及多个步骤,从天然来源提取该化合物开始。 该过程通常包括水解皂苷以获得苷元,然后进行甲基化以生产原薯蓣皂苷元甲酯 . 特定的反应条件,如温度、pH值和催化剂的使用,对于确保合成效率和收率至关重要 .
工业生产方法
原薯蓣皂苷元甲酯的工业生产涉及从薯蓣科植物中大规模提取。 该过程包括使用溶剂提取,然后进行色谱等纯化步骤以分离该化合物 . 生物技术的进步也使通过微生物发酵生产原薯蓣皂苷元甲酯成为可能,这提供了一种更可持续和可扩展的方法 .
化学反应分析
反应类型
原薯蓣皂苷元甲酯经历各种化学反应,包括:
常用试剂和条件
原薯蓣皂苷元甲酯反应中常用的试剂包括氧化剂(例如高锰酸钾)、还原剂(例如硼氢化钠)和烷基化剂(例如碘甲烷) . 温度、压力和pH值等反应条件被仔细控制,以优化反应的收率和选择性 .
形成的主要产物
原薯蓣皂苷元甲酯反应形成的主要产物取决于反应类型和使用的试剂。 例如,氧化反应可能会生成羟基化衍生物,而还原反应可能会生成脱氧化合物 . 取代反应会导致各种烷基化或卤代衍生物 .
相似化合物的比较
属性
IUPAC Name |
(2S,3R,4R,5R,6S)-2-[(2R,3S,4S,5R,6R)-4-hydroxy-2-(hydroxymethyl)-6-[[(1S,2S,4S,6R,7S,8R,9S,12S,13R,16S)-6-methoxy-7,9,13-trimethyl-6-[(3R)-3-methyl-4-[(2R,3R,4S,5S,6R)-3,4,5-trihydroxy-6-(hydroxymethyl)oxan-2-yl]oxybutyl]-5-oxapentacyclo[10.8.0.02,9.04,8.013,18]icos-18-en-16-yl]oxy]-5-[(2S,3R,4R,5R,6S)-3,4,5-trihydroxy-6-methyloxan-2-yl]oxyoxan-3-yl]oxy-6-methyloxane-3,4,5-triol | |
---|---|---|
Source | PubChem | |
URL | https://pubchem.ncbi.nlm.nih.gov | |
Description | Data deposited in or computed by PubChem | |
InChI |
InChI=1S/C52H86O22/c1-21(20-66-46-40(61)39(60)36(57)31(18-53)70-46)10-15-52(65-7)22(2)33-30(74-52)17-29-27-9-8-25-16-26(11-13-50(25,5)28(27)12-14-51(29,33)6)69-49-45(73-48-42(63)38(59)35(56)24(4)68-48)43(64)44(32(19-54)71-49)72-47-41(62)37(58)34(55)23(3)67-47/h8,21-24,26-49,53-64H,9-20H2,1-7H3/t21-,22+,23+,24+,26+,27-,28+,29+,30+,31-,32-,33+,34+,35+,36-,37-,38-,39+,40-,41-,42-,43+,44-,45-,46-,47+,48+,49-,50+,51+,52-/m1/s1 | |
Source | PubChem | |
URL | https://pubchem.ncbi.nlm.nih.gov | |
Description | Data deposited in or computed by PubChem | |
InChI Key |
HSSJYSJXBOCKQM-GVTGEURHSA-N | |
Source | PubChem | |
URL | https://pubchem.ncbi.nlm.nih.gov | |
Description | Data deposited in or computed by PubChem | |
Canonical SMILES |
CC1C2C(CC3C2(CCC4C3CC=C5C4(CCC(C5)OC6C(C(C(C(O6)CO)OC7C(C(C(C(O7)C)O)O)O)O)OC8C(C(C(C(O8)C)O)O)O)C)C)OC1(CCC(C)COC9C(C(C(C(O9)CO)O)O)O)OC | |
Source | PubChem | |
URL | https://pubchem.ncbi.nlm.nih.gov | |
Description | Data deposited in or computed by PubChem | |
Isomeric SMILES |
C[C@H]1[C@H]2[C@H](C[C@@H]3[C@@]2(CC[C@H]4[C@H]3CC=C5[C@@]4(CC[C@@H](C5)O[C@H]6[C@@H]([C@H]([C@@H]([C@H](O6)CO)O[C@H]7[C@@H]([C@@H]([C@H]([C@@H](O7)C)O)O)O)O)O[C@H]8[C@@H]([C@@H]([C@H]([C@@H](O8)C)O)O)O)C)C)O[C@@]1(CC[C@@H](C)CO[C@H]9[C@@H]([C@H]([C@@H]([C@H](O9)CO)O)O)O)OC | |
Source | PubChem | |
URL | https://pubchem.ncbi.nlm.nih.gov | |
Description | Data deposited in or computed by PubChem | |
Molecular Formula |
C52H86O22 | |
Source | PubChem | |
URL | https://pubchem.ncbi.nlm.nih.gov | |
Description | Data deposited in or computed by PubChem | |
DSSTOX Substance ID |
DTXSID301031695 | |
Record name | Methylprotodioscin | |
Source | EPA DSSTox | |
URL | https://comptox.epa.gov/dashboard/DTXSID301031695 | |
Description | DSSTox provides a high quality public chemistry resource for supporting improved predictive toxicology. | |
Molecular Weight |
1063.2 g/mol | |
Source | PubChem | |
URL | https://pubchem.ncbi.nlm.nih.gov | |
Description | Data deposited in or computed by PubChem | |
CAS No. |
54522-52-0 | |
Record name | Methylprotodioscin | |
Source | CAS Common Chemistry | |
URL | https://commonchemistry.cas.org/detail?cas_rn=54522-52-0 | |
Description | CAS Common Chemistry is an open community resource for accessing chemical information. Nearly 500,000 chemical substances from CAS REGISTRY cover areas of community interest, including common and frequently regulated chemicals, and those relevant to high school and undergraduate chemistry classes. This chemical information, curated by our expert scientists, is provided in alignment with our mission as a division of the American Chemical Society. | |
Explanation | The data from CAS Common Chemistry is provided under a CC-BY-NC 4.0 license, unless otherwise stated. | |
Record name | Methylprotodioscin | |
Source | ChemIDplus | |
URL | https://pubchem.ncbi.nlm.nih.gov/substance/?source=chemidplus&sourceid=0054522520 | |
Description | ChemIDplus is a free, web search system that provides access to the structure and nomenclature authority files used for the identification of chemical substances cited in National Library of Medicine (NLM) databases, including the TOXNET system. | |
Record name | Methylprotodioscin | |
Source | EPA DSSTox | |
URL | https://comptox.epa.gov/dashboard/DTXSID301031695 | |
Description | DSSTox provides a high quality public chemistry resource for supporting improved predictive toxicology. | |
Record name | METHYLPROTODIOSCIN | |
Source | FDA Global Substance Registration System (GSRS) | |
URL | https://gsrs.ncats.nih.gov/ginas/app/beta/substances/1VJB6VV6IA | |
Description | The FDA Global Substance Registration System (GSRS) enables the efficient and accurate exchange of information on what substances are in regulated products. Instead of relying on names, which vary across regulatory domains, countries, and regions, the GSRS knowledge base makes it possible for substances to be defined by standardized, scientific descriptions. | |
Explanation | Unless otherwise noted, the contents of the FDA website (www.fda.gov), both text and graphics, are not copyrighted. They are in the public domain and may be republished, reprinted and otherwise used freely by anyone without the need to obtain permission from FDA. Credit to the U.S. Food and Drug Administration as the source is appreciated but not required. | |
A: Methyl protodioscin (MPD) is a natural furostanol saponin found in plants belonging to the Dioscoreaceae family, such as certain species of yams. [] It has been the subject of extensive research for its diverse pharmacological activities, particularly its anti-tumor, anti-inflammatory, and neuroprotective properties. [, , , , ]
A: MPD has a molecular formula of C51H84O22 and a molecular weight of 1085.2 g/mol. []
ANone: The structure of MPD is elucidated using a combination of spectroscopic techniques, primarily Nuclear Magnetic Resonance (NMR) spectroscopy and Mass Spectrometry (MS). [1H-NMR, 13C-NMR, 1H-1H COSY, HMQC, HMBC, and FAB-MS are commonly employed. 29]
ANone: MPD has been shown to induce apoptosis, or programmed cell death, in various cancer cell lines. Studies suggest that MPD triggers apoptosis via multiple pathways, including:
- Caspase Activation: MPD activates caspase-9 and caspase-3, key enzymes involved in the execution phase of apoptosis. []
- Mitochondrial Pathway: MPD disrupts mitochondrial membrane potential, leading to the release of cytochrome c, a crucial step in the intrinsic apoptotic pathway. []
- MAPK Signaling: MPD modulates the activity of mitogen-activated protein kinases (MAPKs), specifically JNK and p38, which are involved in stress-induced apoptosis. []
- Cell Cycle Arrest: MPD induces cell cycle arrest at the G2/M phase, preventing cancer cells from dividing and proliferating. []
ANone: Yes, in vitro and in vivo studies have demonstrated the anticancer potential of MPD against several cancer types, including:
- Osteosarcoma: MPD inhibits cell growth and induces apoptosis in MG-63 human osteosarcoma cells. []
- Lung Cancer: MPD shows growth inhibitory effects, induces G2/M cell cycle arrest, and triggers apoptosis in A549 human lung cancer cells. []
- Prostate Cancer: MPD suppresses proliferation, migration, invasion, and cell cycle progression while inducing apoptosis in DU145 prostate cancer cells. []
- Pancreatic Cancer: MPD has been found to suppress proliferation and induce apoptosis in pancreatic cancer cells, along with inhibiting glycolysis, a process often upregulated in cancer cells. []
A: MPD has been found to decrease cholesterol concentration, potentially by inducing Forkhead box O (FOXO)1, a tumor suppressor protein involved in cholesterol metabolism. [] This cholesterol-lowering effect may disrupt lipid rafts, specialized membrane microdomains enriched in cholesterol and signaling molecules. The disruption of lipid rafts can negatively impact the function of oncogenic signaling pathways, contributing to MPD's anticancer effects. []
A: Studies suggest that MPD, at therapeutic concentrations, does not significantly inhibit the activity of seven major cytochrome P450 enzymes (CYP1A2, CYP2D6, CYP2C9, CYP2C19, CYP2E1, CYP2A6, and CYP3A4) in human liver microsomes. [] This finding implies a low probability of drug-drug interactions between MPD and drugs metabolized by these enzymes.
A: Pharmacokinetic studies in rats have revealed that MPD exhibits dose-dependent pharmacokinetics following intravenous administration. [, ] The compound is rapidly cleared from the plasma, with an elimination half-life ranging from 25.56 to 29.32 minutes. [] MPD appears to follow a one-compartment model after intravenous injection, indicating rapid distribution and elimination. [] A considerable portion of the administered dose is recovered in urine and bile, suggesting these as primary routes of excretion. []
A: Yes, a highly sensitive and specific high-performance liquid chromatography/tandem mass spectrometry (HPLC-MS/MS) assay has been developed and validated for the quantification of MPD in rat plasma. [] This method utilizes methanol-mediated protein precipitation for sample pretreatment and achieves separation on a C18 column.
A: Yes, the developed HPLC-MS/MS method has been validated for its linearity, specificity, accuracy, precision, and reproducibility, demonstrating its suitability for pharmacokinetic studies. []
ANone: MPD has demonstrated additional pharmacological activities, including:
- Anti-inflammatory effects: MPD reduces airway inflammation by inhibiting the production of pro-inflammatory cytokines like IL-6, IL-8, and TNF-α. []
- Cardioprotective effects: MPD has shown protective effects against myocardial infarction in rats. [] It reduces myocardial enzyme levels, lessens infarct size, and improves the heart's antioxidant capacity. [] It also protects cardiomyocytes from anoxia/reoxygenation injury. []
- Anti-thrombotic effects: MPD displays anti-thrombotic effects in rats by inhibiting both in vitro and in vivo thrombosis. [] It prolongs occlusion time and reduces thrombus weight. [] It also possesses blood viscosity-lowering effects. []
- Anti-osteoporotic activity: Studies have shown that MPD and extracts rich in MPD can inhibit bone loss in ovariectomized rats and mice, indicating potential in treating osteoporosis. []
A: Modifications to the MPD structure can significantly impact its biological activity. For example, the introduction of specific sugar moieties or acyl groups can enhance its anti-inflammatory properties. [] Further research is ongoing to fully understand the SAR of MPD and develop more potent and selective analogs.
A: Yes, several synthetic routes to MPD have been reported. These typically involve multi-step procedures starting from diosgenin, a related steroidal sapogenin. [, , ] Improvements in synthetic strategies have led to more efficient and scalable methods for obtaining MPD and its analogs.
ANone: Despite its promising pharmacological activities, there are challenges in translating MPD into a clinically viable drug. These include:
- Low water solubility: MPD's poor water solubility can hinder its formulation and bioavailability. []
ANone: Yes, researchers are exploring various strategies to enhance the therapeutic profile of MPD, such as:
A: The presence and distribution of MPD and related saponins within specific plant families, like Dioscoreaceae and Palmae, provide valuable insights into plant evolution and classification. [] Chemotaxonomic studies based on MPD can contribute to a better understanding of plant biodiversity and the discovery of new bioactive compounds.
A: Xanthine oxidase is an enzyme involved in purine metabolism. Its inhibition can lead to a decrease in uric acid production, making MPD a potential therapeutic candidate for hyperuricemia and gout. [, ]
A: Computational chemistry plays a crucial role in understanding the interactions between MPD and its biological targets, predicting the properties of new analogs, and guiding the design of improved therapeutics. Techniques like molecular docking and molecular dynamics simulations can provide valuable insights into the binding modes, affinities, and potential mechanisms of action of MPD and its derivatives. []
体外研究产品的免责声明和信息
请注意,BenchChem 上展示的所有文章和产品信息仅供信息参考。 BenchChem 上可购买的产品专为体外研究设计,这些研究在生物体外进行。体外研究,源自拉丁语 "in glass",涉及在受控实验室环境中使用细胞或组织进行的实验。重要的是要注意,这些产品没有被归类为药物或药品,他们没有得到 FDA 的批准,用于预防、治疗或治愈任何医疗状况、疾病或疾病。我们必须强调,将这些产品以任何形式引入人类或动物的身体都是法律严格禁止的。遵守这些指南对确保研究和实验的法律和道德标准的符合性至关重要。