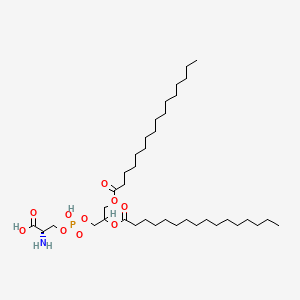
二棕榈酰磷脂酰丝氨酸
概述
描述
Dipalmitoylphosphatidylserine is a phospholipid molecule composed of two palmitic acid chains attached to a phosphatidylserine head group. It is a crucial component of cell membranes, particularly in the inner leaflet of the plasma membrane. This compound plays a significant role in various cellular processes, including apoptosis, blood clotting, and cell signaling.
科学研究应用
Dipalmitoylphosphatidylserine has numerous applications in scientific research, particularly in the fields of chemistry, biology, medicine, and industry. Some key applications include:
Chemistry: Used as a model compound for studying lipid behavior and interactions in membranes.
Biology: Plays a role in cell signaling, apoptosis, and membrane dynamics. It is also used in studies of lipid-protein interactions.
作用机制
Target of Action
Dipalmitoylphosphatidylserine (DPPS) is a phospholipid that primarily targets the plasma membrane of cells . It plays a crucial role in many cellular processes, including apoptotic cell recognition, blood clotting regulation, cellular signaling, and intercellular interactions . DPPS also interacts with specific receptors on immune cells, including macrophages, to improve nanoparticle targeting through an efferocytosis-like mechanism .
Mode of Action
DPPS interacts with its targets through intricate intermolecular interactions. In mixed phosphatidylserine/phosphatidylcholine (PS/PC) bilayers, which serve as a simplified model of the outer leaflets of mammalian cell plasma membranes, DPPS and PC lipids exhibit mutual miscibility . The disparities in the position and orientation of PC and PS headgroups are due to strong electrostatic interactions between like-lipid pairs . Notably, PS headgroups profoundly affect the ordering of the lipid acyl chains, leading to lipid elongation and subtle PS protrusion above the zwitterionic membrane .
Biochemical Pathways
DPPS is involved in several biochemical pathways. It is biosynthesized via a de novo pathway, the last step of which is catalyzed by CDP-choline:cholinephosphotransferase (CPT). This process also involves two remodeling steps: a deacylation and a reacylation one, catalyzed by an acidic, Ca2±independent phospholipase A2 and a lyso-phosphatidylcholine acyltransferase, respectively . These pathways are crucial for the biosynthesis of phosphatidylcholine and its remodeling to DPPS in lung epithelial cells .
Pharmacokinetics
It’s known that dpps-filled cationic maltodextrin nanoparticles exhibit enhanced efficacy for cell entry and intracellular protein delivery in phagocytic thp-1 cells .
Result of Action
The interaction of DPPS with the plasma membrane results in several molecular and cellular effects. For instance, DPPS-filled cationic maltodextrin nanoparticles enter cells faster and deliver more proteins than their counterparts . Moreover, DPPS profoundly affects the ordering of the lipid acyl chains, leading to lipid elongation .
Action Environment
The action of DPPS is influenced by various environmental factors. For instance, the lipid phase state of the membrane affects the interaction of DPPS with the membrane . Moreover, the presence of cholesterol in the plasma membrane can reduce the efficacy of DPPS . These factors can modulate the action, efficacy, and stability of DPPS.
未来方向
Future research on DPPS could focus on its potential applications in drug delivery, as suggested by the study on DPPS-filled cationic maltodextrin nanoparticles . Another interesting direction could be the investigation of the interactions of DPPS with various drugs, as in the study with the antimalarial drug amodiaquine .
生化分析
Biochemical Properties
Dipalmitoylphosphatidylserine is involved in several biochemical reactions, primarily due to its role as a phospholipid in cell membranes. It interacts with various enzymes, proteins, and other biomolecules. For instance, it is known to interact with protein kinase C, which is activated by the binding of dipalmitoylphosphatidylserine to its regulatory domain . This interaction is crucial for the enzyme’s role in signal transduction pathways. Additionally, dipalmitoylphosphatidylserine interacts with annexin V, a protein that binds to phosphatidylserine in a calcium-dependent manner, playing a role in the regulation of apoptosis .
Cellular Effects
Dipalmitoylphosphatidylserine has significant effects on various cell types and cellular processes. It influences cell function by affecting cell signaling pathways, gene expression, and cellular metabolism. For example, the exposure of dipalmitoylphosphatidylserine on the outer leaflet of the plasma membrane is a signal for phagocytosis of apoptotic cells . This process is essential for maintaining cellular homeostasis and preventing inflammation. Furthermore, dipalmitoylphosphatidylserine has been shown to modulate the activity of ion channels and receptors, thereby influencing cellular signaling pathways .
Molecular Mechanism
At the molecular level, dipalmitoylphosphatidylserine exerts its effects through various mechanisms. It binds to specific proteins, such as protein kinase C and annexin V, influencing their activity and function . Additionally, dipalmitoylphosphatidylserine can modulate the activity of enzymes involved in lipid metabolism, such as phospholipase A2, by altering the membrane’s physical properties . This modulation can lead to changes in gene expression and cellular responses to external stimuli.
Temporal Effects in Laboratory Settings
In laboratory settings, the effects of dipalmitoylphosphatidylserine can change over time. The stability and degradation of dipalmitoylphosphatidylserine are influenced by factors such as temperature, pH, and the presence of enzymes . Long-term studies have shown that dipalmitoylphosphatidylserine can affect cellular function over extended periods, with potential implications for cell viability and function . For example, prolonged exposure to dipalmitoylphosphatidylserine can lead to changes in membrane fluidity and the activation of signaling pathways involved in cell survival and apoptosis .
Dosage Effects in Animal Models
The effects of dipalmitoylphosphatidylserine vary with different dosages in animal models. At low doses, dipalmitoylphosphatidylserine can enhance cellular function and promote cell survival . At high doses, it may induce toxic effects, such as membrane destabilization and the activation of apoptotic pathways . Studies in animal models have shown that there is a threshold dose beyond which the beneficial effects of dipalmitoylphosphatidylserine are outweighed by its toxic effects .
Metabolic Pathways
Dipalmitoylphosphatidylserine is involved in several metabolic pathways, including those related to lipid metabolism and cell signaling . It interacts with enzymes such as phosphatidylserine synthase, which catalyzes its synthesis from phosphatidylcholine and serine . Additionally, dipalmitoylphosphatidylserine can influence metabolic flux by modulating the activity of enzymes involved in lipid degradation and synthesis . This modulation can lead to changes in metabolite levels and cellular responses to metabolic stress .
Transport and Distribution
Within cells and tissues, dipalmitoylphosphatidylserine is transported and distributed through various mechanisms . It interacts with transporters and binding proteins that facilitate its movement across cellular membranes . For example, dipalmitoylphosphatidylserine can be transported to the plasma membrane via vesicular transport pathways . Its distribution within cells is also influenced by its interactions with other lipids and proteins, which can affect its localization and accumulation .
Subcellular Localization
Dipalmitoylphosphatidylserine is localized to specific subcellular compartments, where it exerts its activity and function . It is primarily found in the inner leaflet of the plasma membrane, but it can also be localized to other organelles, such as the endoplasmic reticulum and mitochondria . The subcellular localization of dipalmitoylphosphatidylserine is regulated by targeting signals and post-translational modifications that direct it to specific compartments . These localization patterns are crucial for its role in cellular signaling and membrane dynamics .
准备方法
Synthetic Routes and Reaction Conditions
Dipalmitoylphosphatidylserine can be synthesized through several methods. One common approach is the thin-film hydration method, where a lipid film is hydrated with an aqueous buffer to form liposomes. The process involves dissolving the lipid in an organic solvent, evaporating the solvent to form a thin film, and then hydrating the film with an aqueous buffer under controlled conditions .
Another method involves the use of microemulsification, sonication, membrane extrusion, freeze-thawing, ether injection, and ethanol injection . These methods vary in terms of efficiency, scalability, and the physical properties of the resulting liposomes.
Industrial Production Methods
Industrial production of dipalmitoylphosphatidylserine typically involves large-scale synthesis using automated systems to ensure consistency and purity. The process includes the synthesis of the lipid, purification, and formulation into liposomes or other delivery systems. Quality control measures are implemented to ensure the final product meets the required specifications for pharmaceutical or research applications.
化学反应分析
Types of Reactions
Dipalmitoylphosphatidylserine undergoes various chemical reactions, including oxidation, reduction, and substitution. These reactions can alter the physical and chemical properties of the compound, affecting its functionality in biological systems.
Common Reagents and Conditions
Oxidation: Common oxidizing agents include hydrogen peroxide and molecular oxygen. These reactions typically occur under mild conditions and can lead to the formation of oxidized lipid species.
Reduction: Reducing agents such as sodium borohydride or lithium aluminum hydride can be used to reduce oxidized lipid species back to their original form.
Substitution: Substitution reactions often involve the exchange of functional groups on the phosphatidylserine head group. Common reagents include alkylating agents and nucleophiles.
Major Products Formed
The major products formed from these reactions depend on the specific conditions and reagents used. For example, oxidation can lead to the formation of lipid peroxides, while reduction can restore the original lipid structure. Substitution reactions can result in modified phosphatidylserine derivatives with altered functional properties.
相似化合物的比较
Similar Compounds
Dipalmitoylphosphatidylcholine: Another phospholipid with two palmitic acid chains, but with a phosphatidylcholine head group.
Phosphatidylethanolamine: A phospholipid with an ethanolamine head group, involved in membrane fusion and cell signaling.
Phosphatidylinositol: A phospholipid with an inositol head group, important in cell signaling and membrane trafficking.
Uniqueness
Dipalmitoylphosphatidylserine is unique due to its specific head group, which imparts distinct functional properties. It is particularly important in apoptosis, where its externalization on the cell membrane serves as a signal for phagocytic cells to engulf and remove apoptotic cells. This property distinguishes it from other phospholipids, which do not have the same signaling role in apoptosis .
属性
IUPAC Name |
2-amino-3-[2,3-di(hexadecanoyloxy)propoxy-hydroxyphosphoryl]oxypropanoic acid | |
---|---|---|
Details | Computed by Lexichem TK 2.7.0 (PubChem release 2021.05.07) | |
Source | PubChem | |
URL | https://pubchem.ncbi.nlm.nih.gov | |
Description | Data deposited in or computed by PubChem | |
InChI |
InChI=1S/C38H74NO10P/c1-3-5-7-9-11-13-15-17-19-21-23-25-27-29-36(40)46-31-34(32-47-50(44,45)48-33-35(39)38(42)43)49-37(41)30-28-26-24-22-20-18-16-14-12-10-8-6-4-2/h34-35H,3-33,39H2,1-2H3,(H,42,43)(H,44,45) | |
Details | Computed by InChI 1.0.6 (PubChem release 2021.05.07) | |
Source | PubChem | |
URL | https://pubchem.ncbi.nlm.nih.gov | |
Description | Data deposited in or computed by PubChem | |
InChI Key |
KLFKZIQAIPDJCW-UHFFFAOYSA-N | |
Details | Computed by InChI 1.0.6 (PubChem release 2021.05.07) | |
Source | PubChem | |
URL | https://pubchem.ncbi.nlm.nih.gov | |
Description | Data deposited in or computed by PubChem | |
Canonical SMILES |
CCCCCCCCCCCCCCCC(=O)OCC(COP(=O)(O)OCC(C(=O)O)N)OC(=O)CCCCCCCCCCCCCCC | |
Details | Computed by OEChem 2.3.0 (PubChem release 2021.05.07) | |
Source | PubChem | |
URL | https://pubchem.ncbi.nlm.nih.gov | |
Description | Data deposited in or computed by PubChem | |
Molecular Formula |
C38H74NO10P | |
Details | Computed by PubChem 2.1 (PubChem release 2021.05.07) | |
Source | PubChem | |
URL | https://pubchem.ncbi.nlm.nih.gov | |
Description | Data deposited in or computed by PubChem | |
DSSTOX Substance ID |
DTXSID90863085 | |
Record name | O-{[2,3-Bis(hexadecanoyloxy)propoxy](hydroxy)phosphoryl}serine | |
Source | EPA DSSTox | |
URL | https://comptox.epa.gov/dashboard/DTXSID90863085 | |
Description | DSSTox provides a high quality public chemistry resource for supporting improved predictive toxicology. | |
Molecular Weight |
736.0 g/mol | |
Details | Computed by PubChem 2.1 (PubChem release 2021.05.07) | |
Source | PubChem | |
URL | https://pubchem.ncbi.nlm.nih.gov | |
Description | Data deposited in or computed by PubChem | |
CAS No. |
40290-43-5 | |
Record name | DL-Dipalmitoylphosphatidylserine | |
Source | ChemIDplus | |
URL | https://pubchem.ncbi.nlm.nih.gov/substance/?source=chemidplus&sourceid=0040290435 | |
Description | ChemIDplus is a free, web search system that provides access to the structure and nomenclature authority files used for the identification of chemical substances cited in National Library of Medicine (NLM) databases, including the TOXNET system. | |
Retrosynthesis Analysis
AI-Powered Synthesis Planning: Our tool employs the Template_relevance Pistachio, Template_relevance Bkms_metabolic, Template_relevance Pistachio_ringbreaker, Template_relevance Reaxys, Template_relevance Reaxys_biocatalysis model, leveraging a vast database of chemical reactions to predict feasible synthetic routes.
One-Step Synthesis Focus: Specifically designed for one-step synthesis, it provides concise and direct routes for your target compounds, streamlining the synthesis process.
Accurate Predictions: Utilizing the extensive PISTACHIO, BKMS_METABOLIC, PISTACHIO_RINGBREAKER, REAXYS, REAXYS_BIOCATALYSIS database, our tool offers high-accuracy predictions, reflecting the latest in chemical research and data.
Strategy Settings
Precursor scoring | Relevance Heuristic |
---|---|
Min. plausibility | 0.01 |
Model | Template_relevance |
Template Set | Pistachio/Bkms_metabolic/Pistachio_ringbreaker/Reaxys/Reaxys_biocatalysis |
Top-N result to add to graph | 6 |
Feasible Synthetic Routes
Q1: What is the molecular structure and formula of Dipalmitoylphosphatidylserine (DPPS)?
A1: Dipalmitoylphosphatidylserine consists of a glycerol backbone esterified to two palmitic acid molecules (saturated fatty acids with 16 carbon atoms) and a phosphoserine headgroup. Its molecular formula is C41H82NO10P.
Q2: Does the ionization state of DPPS change with pH?
A2: Yes. Dipalmitoylphosphatidylserine carries a net negative charge at neutral pH due to the ionization of the carboxyl and phosphate groups in the serine headgroup. [] The apparent pKa (pH at 50% ionization) of the carboxyl group in the crystalline form of DPPS shifts from 6.0 to 4.6 to 3.7 as the NaCl concentration increases from 10^-3 to 0.1 to 1.0 M. []
Q3: How does the phase transition temperature of DPPS change with pH?
A3: The gel-to-fluid phase transition temperature of DPPS bilayers is highly sensitive to pH. Protonation of the carboxyl group (around pH 5.5) increases the transition temperature from 54 °C to 62 °C in fully hydrated DPPS. Deprotonation of the amino group (around pH 11.55) decreases the transition temperature to approximately 32 °C. []
Q4: What techniques are used to study the phase transitions of DPPS?
A4: Differential scanning calorimetry (DSC) is widely employed to study the phase transitions of DPPS bilayers. This technique allows researchers to determine the transition temperature and enthalpy changes associated with the gel-to-fluid transition. [, , , , ]
Q5: What is the effect of salt concentration on the phase transition temperature of DPPS?
A5: Increasing salt (NaCl) concentration generally increases the transition temperature of DPPS. This effect is attributed to the screening of electrostatic interactions between the negatively charged headgroups, promoting a more ordered packing of the lipids. At higher salt concentrations, ion binding and displacement of water molecules further contribute to the increase in transition temperature. []
Q6: How does DPPS interact with divalent cations like calcium (Ca2+)?
A6: Dipalmitoylphosphatidylserine exhibits a strong affinity for divalent cations like Ca2+. This interaction leads to the formation of DPPS-Ca2+ complexes, which are crucial for various biological processes, including blood coagulation and membrane fusion. [, , ] The presence of Ca2+ induces a phase transition in DPPS monolayers, shifting them from a liquid-expanded to a condensed phase. []
Q7: Can you elaborate on the role of DPPS in blood coagulation?
A7: Dipalmitoylphosphatidylserine plays a vital role in blood coagulation by providing a negatively charged surface for the binding of vitamin K-dependent clotting factors, such as prothrombin and factor Xa. This binding event, mediated by calcium ions, is essential for the activation of these factors and subsequent fibrin clot formation. [, , ]
Q8: How does DPPS influence the activity of antimicrobial peptides like surfactin?
A8: The interaction of surfactin, a cyclic lipopeptide, with DPPS monolayers is influenced by the electrostatic interactions between the negatively charged DPPS headgroups and the peptide. These interactions can modulate the penetration of surfactin into the monolayer and its ability to alter the nanoscale organization of the lipid film. []
Q9: What are the potential applications of DPPS in drug delivery?
A10: Dipalmitoylphosphatidylserine has been explored as a component of liposomal drug delivery systems. Its negative charge can facilitate the encapsulation of positively charged drugs, and its ability to interact with cell membranes may enhance drug uptake by target cells. [, , ]
Q10: Are there specific challenges associated with using DPPS in drug delivery formulations?
A11: One challenge in utilizing DPPS for drug delivery is its potential to induce aggregation of liposomes due to inter-liposomal interactions between the negatively charged headgroups. This aggregation can affect the stability and drug release properties of the liposomal formulation. []
Q11: Can you provide an example of how DPPS has been used to enhance drug delivery?
A12: Dipalmitoylphosphatidylserine has been incorporated into liposomal formulations of the antiviral drug ofloxacin. This encapsulation strategy led to increased drug accumulation within bacteria and enhanced antimicrobial activity compared to the free drug. []
体外研究产品的免责声明和信息
请注意,BenchChem 上展示的所有文章和产品信息仅供信息参考。 BenchChem 上可购买的产品专为体外研究设计,这些研究在生物体外进行。体外研究,源自拉丁语 "in glass",涉及在受控实验室环境中使用细胞或组织进行的实验。重要的是要注意,这些产品没有被归类为药物或药品,他们没有得到 FDA 的批准,用于预防、治疗或治愈任何医疗状况、疾病或疾病。我们必须强调,将这些产品以任何形式引入人类或动物的身体都是法律严格禁止的。遵守这些指南对确保研究和实验的法律和道德标准的符合性至关重要。