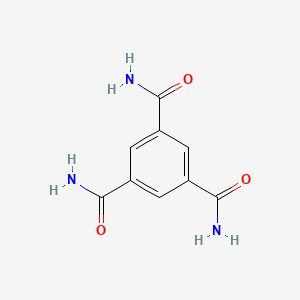
苯-1,3,5-三甲酰胺
描述
Benzene-1,3,5-tricarboxamide (BTA) is a versatile ordering moiety for supramolecular chemistry . Its simple structure and wide accessibility, combined with a detailed understanding of its supramolecular self-assembly behavior, allow full utilization of this versatile, supramolecular building block in applications ranging from nanotechnology to polymer processing and biomedical applications .
Synthesis Analysis
BTAs were first synthesized in 1915 by Curtius . Their synthesis involves the use of commercially available 5-aminoisophthalic acid . This approach eliminates the statistical reactions and reduces the number of synthetic steps .
Molecular Structure Analysis
The opportunities in the former cases are connected to the self-assembly of BTAs into one-dimensional, nanometer-sized rod-like structures stabilized by threefold H-bonding . Their multivalent nature drives applications in the biomedical field .
Chemical Reactions Analysis
BTAs have been shown to form functional soft-materials by virtue of their complementary hydrogen bonding . They are explored as structurally reinforcing supramolecular building blocks in porous coordination polymers .
Physical And Chemical Properties Analysis
BTAs have a simple structure and wide accessibility . They are known for their tendencies to form functional soft-materials by virtue of their complementary hydrogen bonding .
科学研究应用
Supramolecular Chemistry
BTA has been identified as a versatile ordering moiety for supramolecular chemistry . Its simple structure and wide accessibility, combined with a detailed understanding of its supramolecular self-assembly behavior, allow full utilization of this versatile, supramolecular building block .
Nanotechnology
In the field of nanotechnology, BTAs are used due to their ability to self-assemble into one-dimensional, nanometer-sized rod-like structures stabilized by threefold H-bonding . This property makes them ideal for creating nanostructures with specific properties.
Polymer Processing
BTAs are also used in polymer processing. Their self-assembly behavior allows them to be used as building blocks in the creation of complex polymer structures . This can lead to polymers with unique properties that can be tailored for specific applications.
Biomedical Applications
The multivalent nature of BTAs drives applications in the biomedical field . They can be used to create functional multicomponent biomaterials . Their morphologies and intrinsic dynamic behavior mimic fibrous structures found in nature, making them uniquely suited for biomedical applications .
Functional Multicomponent Biomaterials
BTAs have attracted a lot of interest in recent years as they are uniquely suited to generate functional multicomponent biomaterials . Their modularity allows control of the density of functionalities presented on the surface of the fibers when using functionalized BTA monomers .
Supramolecular Polymers
BTAs are used to create synthetic water-compatible supramolecular polymers . These polymers have a morphology and dynamic behavior similar to BTA-homopolymers . They can be decorated with a fluorescent dye to visualize their co-assembly with BTAs .
作用机制
Target of Action
Benzene-1,3,5-tricarboxamide (BTA) is a versatile molecule that primarily targets the formation of supramolecular structures . Its simple structure and wide accessibility, combined with a detailed understanding of its supramolecular self-assembly behavior, allow full utilization of this versatile, supramolecular building block in applications ranging from nanotechnology to polymer processing and biomedical applications .
Mode of Action
The mode of action of BTA is primarily through the self-assembly into one-dimensional, nanometer-sized rod-like structures stabilized by threefold H-bonding . This self-assembly is driven by the multivalent nature of BTAs, which is particularly useful in the biomedical field .
Biochemical Pathways
The biochemical pathways affected by BTAs are related to their ability to form functional soft-materials by virtue of their complementary hydrogen bonding . They are explored as structurally reinforcing supramolecular building blocks in porous coordination polymers .
Pharmacokinetics
Their solubility and self-assembly behavior are critical factors influencing their bioavailability . For instance, inversed amide BTAs (iBTAs) form intermolecular hydrogen bonds and assemble into supramolecular polymers, albeit with a slight decrease in water solubility .
Result of Action
The result of BTA’s action is the formation of supramolecular structures that mimic fibrous structures found in nature . These structures can be used to generate functional multicomponent biomaterials . For instance, BTAs have been used to create scaffolds for cartilage and bone regeneration, targeted therapy for atherosclerosis, and the promotion of angiogenesis .
Action Environment
The action of BTAs can be influenced by environmental factors. For example, the dynamic properties of BTAs can be tuned by mixing mono- and bivalent variants . This allows the control of the number of biological recognition motifs presented on the supramolecular structures by mixing in different functional monomers in the desired ratio .
安全和危害
未来方向
BTAs have attracted a lot of interest in recent years, as they are uniquely suited to generate functional multicomponent biomaterials . Their morphologies and intrinsic dynamic behavior mimic fibrous structures found in nature . Currently, the first commercial applications of BTAs are emerging . The adaptable nature of this multipurpose building block promises a bright future .
属性
IUPAC Name |
benzene-1,3,5-tricarboxamide | |
---|---|---|
Source | PubChem | |
URL | https://pubchem.ncbi.nlm.nih.gov | |
Description | Data deposited in or computed by PubChem | |
InChI |
InChI=1S/C9H9N3O3/c10-7(13)4-1-5(8(11)14)3-6(2-4)9(12)15/h1-3H,(H2,10,13)(H2,11,14)(H2,12,15) | |
Source | PubChem | |
URL | https://pubchem.ncbi.nlm.nih.gov | |
Description | Data deposited in or computed by PubChem | |
InChI Key |
WXWQVSOHWXJBDF-UHFFFAOYSA-N | |
Source | PubChem | |
URL | https://pubchem.ncbi.nlm.nih.gov | |
Description | Data deposited in or computed by PubChem | |
Canonical SMILES |
C1=C(C=C(C=C1C(=O)N)C(=O)N)C(=O)N | |
Source | PubChem | |
URL | https://pubchem.ncbi.nlm.nih.gov | |
Description | Data deposited in or computed by PubChem | |
Molecular Formula |
C9H9N3O3 | |
Source | PubChem | |
URL | https://pubchem.ncbi.nlm.nih.gov | |
Description | Data deposited in or computed by PubChem | |
DSSTOX Substance ID |
DTXSID90332281 | |
Record name | 1,3,5-Benzenetricarboxamide | |
Source | EPA DSSTox | |
URL | https://comptox.epa.gov/dashboard/DTXSID90332281 | |
Description | DSSTox provides a high quality public chemistry resource for supporting improved predictive toxicology. | |
Molecular Weight |
207.19 g/mol | |
Source | PubChem | |
URL | https://pubchem.ncbi.nlm.nih.gov | |
Description | Data deposited in or computed by PubChem | |
Product Name |
Benzene-1,3,5-tricarboxamide | |
CAS RN |
60541-32-4 | |
Record name | 1,3,5-Benzenetricarboxamide | |
Source | CAS Common Chemistry | |
URL | https://commonchemistry.cas.org/detail?cas_rn=60541-32-4 | |
Description | CAS Common Chemistry is an open community resource for accessing chemical information. Nearly 500,000 chemical substances from CAS REGISTRY cover areas of community interest, including common and frequently regulated chemicals, and those relevant to high school and undergraduate chemistry classes. This chemical information, curated by our expert scientists, is provided in alignment with our mission as a division of the American Chemical Society. | |
Explanation | The data from CAS Common Chemistry is provided under a CC-BY-NC 4.0 license, unless otherwise stated. | |
Record name | 1,3,5-Benzenetricarboxamide | |
Source | EPA DSSTox | |
URL | https://comptox.epa.gov/dashboard/DTXSID90332281 | |
Description | DSSTox provides a high quality public chemistry resource for supporting improved predictive toxicology. | |
Retrosynthesis Analysis
AI-Powered Synthesis Planning: Our tool employs the Template_relevance Pistachio, Template_relevance Bkms_metabolic, Template_relevance Pistachio_ringbreaker, Template_relevance Reaxys, Template_relevance Reaxys_biocatalysis model, leveraging a vast database of chemical reactions to predict feasible synthetic routes.
One-Step Synthesis Focus: Specifically designed for one-step synthesis, it provides concise and direct routes for your target compounds, streamlining the synthesis process.
Accurate Predictions: Utilizing the extensive PISTACHIO, BKMS_METABOLIC, PISTACHIO_RINGBREAKER, REAXYS, REAXYS_BIOCATALYSIS database, our tool offers high-accuracy predictions, reflecting the latest in chemical research and data.
Strategy Settings
Precursor scoring | Relevance Heuristic |
---|---|
Min. plausibility | 0.01 |
Model | Template_relevance |
Template Set | Pistachio/Bkms_metabolic/Pistachio_ringbreaker/Reaxys/Reaxys_biocatalysis |
Top-N result to add to graph | 6 |
Feasible Synthetic Routes
Q & A
Q1: What is the primary driving force for BTA self-assembly?
A: The primary driving force behind BTA self-assembly is the formation of strong, directional threefold α-helical type intermolecular hydrogen bonds between adjacent BTA molecules. [, , ] This leads to the formation of one-dimensional columnar aggregates, often described as helical stacks or nanorods. [, , , , ]
Q2: How does the chirality of BTA monomers influence the self-assembly process?
A: Introducing a stereogenic center into the alkyl side chains of BTAs leads to a preference for one helical conformation over the other, evident in the strong Cotton effects observed in dilute apolar solutions. [, , , ] This chiral amplification arises from the energetic preference of homochiral interactions over heterochiral ones during assembly.
Q3: What types of higher-order structures can BTAs form?
A3: Beyond one-dimensional aggregates, BTAs can assemble into more complex architectures. For instance, they can form:
- Twisted nanofibers: Driven by interfiber interactions and influenced by the peptide sequence in BTA-peptide conjugates. []
- Organogels: Formed in various nonpolar solvents, exhibiting viscoelastic properties dependent on BTA concentration and solvent type. [, , ]
- Membranes and nanotubes: Observed with carboxylic acid functionalized, water-soluble BTAs, showcasing the system's ability to adopt polymorphic structures. []
Q4: How does solvent polarity affect BTA self-assembly?
A: BTA self-assembly is highly sensitive to solvent polarity. Increasing the solvent polarity disrupts the hydrogen bonding network, leading to a decrease in aggregate stability. [, ] This effect is observed both in dilute solutions and in solid-state materials where BTAs are incorporated into polymers. []
Q5: How do the alkyl side chains of BTAs impact their self-assembly?
A5: The length and branching of alkyl side chains significantly impact BTA self-assembly:
- Chain Length: Increasing the chain length generally enhances the stability of aggregates due to stronger van der Waals interactions between side chains. []
- Branching: Branching can influence the packing arrangements and liquid crystalline behavior. For example, branched 3,7-dimethyloctanoyl chains promote the formation of liquid crystalline phases, while linear chains might favor crystalline structures. []
Q6: Can the hydrogen bonding pattern in BTAs be modified?
A: Yes, modifications to the BTA core can alter the hydrogen bonding pattern. For instance, amino ester-based BTAs exhibit both amide-amide (AA) and amide-carboxylate (AC) hydrogen bonding. [] While AC dimers are more thermodynamically stable, AA interactions are crucial for the formation of extended supramolecular polymers. []
Q7: What are some notable properties of BTA-based materials?
A7: BTA-based materials exhibit a range of interesting properties, including:
- Thermotropic liquid crystallinity: Observed in several BTA derivatives, with the mesophase stability and range influenced by side chain structure and intermolecular interactions. [, ]
- Viscoelasticity: BTA organogels demonstrate viscoelastic behavior, with properties tunable by varying BTA concentration, solvent, and temperature. [, ]
- Polar switching: Hydrogen-bonded hexagonal columnar liquid crystal phases formed by BTAs can be aligned by electric fields, displaying polar switching behavior with potential applications in electronic devices. []
- Fluorescence: BTA derivatives incorporating fluorophores like cyanostilbene exhibit aggregation-induced emission enhancement (AIEE), with fluorescence properties tunable by controlling the self-assembly process. []
Q8: What are the potential applications of BTAs in materials science?
A8: The unique self-assembly and stimuli-responsive properties of BTAs make them attractive candidates for various applications, including:
- Electro-optic devices: BTAs that form liquid crystalline phases with polar switching behavior show promise for use in displays, sensors, and other electro-optic applications. [, ]
- Organogels and soft materials: BTA-based organogels, with their tunable viscoelastic properties, can be used in areas like drug delivery, cosmetics, and sensors. [, ]
- Biomimetic materials: The fibrous nature of BTA assemblies and their ability to incorporate biomolecules make them promising for developing biocompatible scaffolds for tissue engineering and drug delivery. [, ]
Q9: How have computational methods been used to study BTA self-assembly?
A9: Computational chemistry plays a crucial role in understanding and predicting BTA self-assembly. Techniques like:
- Density functional theory (DFT): Used to investigate the strength and geometry of hydrogen bonding interactions, providing insights into the driving forces behind BTA self-assembly and the impact of structural modifications. [, , , ]
- Molecular dynamics (MD) simulations: Employed to simulate the self-assembly process in realistic solvents, providing information on aggregate stability, dynamics, and the influence of solvent on assembly. [, , ]
Q10: What is the significance of the cooperativity factor (σ) in BTA self-assembly?
A: The cooperativity factor quantifies the extent of cooperativity in the self-assembly process. BTAs exhibit a wide range of σ values, from isodesmic (σ = 1) to highly cooperative (σ < 10-6). [] Understanding the relationship between BTA structure and σ allows for the rational design of BTA derivatives with tailored self-assembly properties. []
Q11: How does the amide connectivity in BTAs influence their properties?
A: The amide connectivity significantly affects BTA self-assembly. While conventionally synthesized BTAs have carbonyl (C=O)-centered amides, N-centered BTAs (N-BTAs) show weaker aggregation and less pronounced chiral amplification. [] DFT calculations revealed that this difference stems from the lower interaction energy between monomeric units in N-BTA supramolecular polymers. []
体外研究产品的免责声明和信息
请注意,BenchChem 上展示的所有文章和产品信息仅供信息参考。 BenchChem 上可购买的产品专为体外研究设计,这些研究在生物体外进行。体外研究,源自拉丁语 "in glass",涉及在受控实验室环境中使用细胞或组织进行的实验。重要的是要注意,这些产品没有被归类为药物或药品,他们没有得到 FDA 的批准,用于预防、治疗或治愈任何医疗状况、疾病或疾病。我们必须强调,将这些产品以任何形式引入人类或动物的身体都是法律严格禁止的。遵守这些指南对确保研究和实验的法律和道德标准的符合性至关重要。