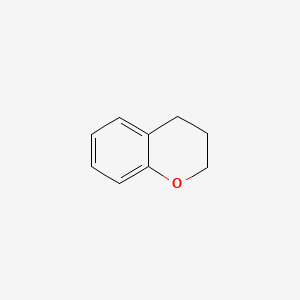
Chromane
概述
描述
Chromane is a benzopyran consisting of a pyran ring having a benzene ring ortho-fused across positions 2 and 3.
作用机制
Target of Action
Chroman, also known as Chromane, is a heterobicyclic compound that acts as a building block in medicinal chemistry for the isolation, design, and synthesis of novel lead compounds . It has been found to target several biological entities, including tumor necrosis factor-α (TNF-α), which plays a significant role in the pathological mechanism of various inflammation-related diseases . Another target of Chroman derivatives is Pteridine Reductase 1 (PTR1), an enzyme involved in parasitic infections .
Mode of Action
Chroman interacts with its targets in a variety of ways. For instance, it inhibits the TNF-α-induced expression of ICAM-1 on endothelial cells . In the case of PTR1, Chroman derivatives bind to the enzyme, inhibiting its function and showing anti-parasitic activity . The specific interactions between Chroman and its targets can vary depending on the structure of the Chroman derivative and the nature of the target.
Biochemical Pathways
Chroman affects several biochemical pathways due to its diverse biological activities. It has been associated with anti-inflammatory, antivascular, antidiabetic, antioxidant, antimicrobial, antifungal, antiviral, antileishmanial, and antitubercular activity . The exact pathways affected can depend on the specific targets of the Chroman derivative and the biological context in which it is acting.
Pharmacokinetics
The Absorption, Distribution, Metabolism, and Excretion (ADME) properties of Chroman are crucial for its bioavailability and therapeutic effectiveness
Result of Action
The molecular and cellular effects of Chroman’s action are diverse due to its wide range of biological activities. For instance, it has been found to inhibit the expression of ICAM-1 on endothelial cells, which can reduce inflammation . Additionally, its anti-parasitic activity results from the inhibition of PTR1 . These effects can vary depending on the specific Chroman derivative and the biological context.
生化分析
Biochemical Properties
Chromane plays a crucial role in biochemical reactions. It interacts with several enzymes, proteins, and other biomolecules. For instance, this compound derivatives have been found to inhibit protein kinases, aldose reductase, and HIV-1 reverse transcriptase . These interactions are typically characterized by the binding of this compound to the active sites of these enzymes, leading to inhibition of their activity. Additionally, this compound exhibits antioxidant properties, which contribute to its biochemical significance.
Cellular Effects
This compound influences various cellular processes and functions. It has been shown to affect cell signaling pathways, gene expression, and cellular metabolism. For example, this compound derivatives can modulate the activity of signaling molecules such as kinases, thereby influencing cell proliferation and apoptosis . Furthermore, this compound can alter gene expression patterns, leading to changes in cellular behavior and function. Its impact on cellular metabolism includes the regulation of oxidative stress and energy production.
Molecular Mechanism
The molecular mechanism of this compound involves its interaction with biomolecules at the molecular level. This compound binds to specific sites on enzymes and proteins, leading to inhibition or activation of their activity. For instance, this compound derivatives inhibit aldose reductase by binding to its active site, preventing the conversion of glucose to sorbitol . Additionally, this compound can influence gene expression by interacting with transcription factors and other regulatory proteins, thereby modulating the transcription of target genes.
Temporal Effects in Laboratory Settings
In laboratory settings, the effects of this compound can change over time. This compound is generally stable under standard laboratory conditions, but it can degrade under extreme conditions such as high temperature or acidic pH. Long-term studies have shown that this compound can have sustained effects on cellular function, including prolonged inhibition of enzyme activity and modulation of gene expression . These temporal effects are crucial for understanding the long-term impact of this compound in biochemical research.
Dosage Effects in Animal Models
The effects of this compound vary with different dosages in animal models. At low doses, this compound exhibits beneficial effects such as antioxidant activity and enzyme inhibition. At high doses, this compound can exhibit toxic effects, including cellular damage and disruption of normal cellular functions . Threshold effects have been observed, where a certain dosage level is required to achieve the desired biochemical effect. Understanding the dosage effects of this compound is essential for its safe and effective use in research and therapeutic applications.
Metabolic Pathways
This compound is involved in several metabolic pathways. It interacts with enzymes and cofactors that are crucial for its metabolism. For instance, this compound derivatives are metabolized by cytochrome P450 enzymes, leading to the formation of various metabolites . These metabolic pathways can influence the overall bioavailability and activity of this compound in biological systems. Additionally, this compound can affect metabolic flux and metabolite levels, contributing to its biochemical significance.
Transport and Distribution
This compound is transported and distributed within cells and tissues through specific transporters and binding proteins. These interactions influence the localization and accumulation of this compound in different cellular compartments . For example, this compound can bind to plasma proteins, facilitating its transport in the bloodstream. Understanding the transport and distribution of this compound is crucial for elucidating its biochemical effects and therapeutic potential.
Subcellular Localization
The subcellular localization of this compound is essential for its activity and function. This compound can be directed to specific cellular compartments or organelles through targeting signals and post-translational modifications . For instance, this compound derivatives can localize to the mitochondria, where they exert their antioxidant effects. The subcellular localization of this compound is a key factor in determining its biochemical properties and therapeutic potential.
属性
IUPAC Name |
3,4-dihydro-2H-chromene | |
---|---|---|
Source | PubChem | |
URL | https://pubchem.ncbi.nlm.nih.gov | |
Description | Data deposited in or computed by PubChem | |
InChI |
InChI=1S/C9H10O/c1-2-6-9-8(4-1)5-3-7-10-9/h1-2,4,6H,3,5,7H2 | |
Source | PubChem | |
URL | https://pubchem.ncbi.nlm.nih.gov | |
Description | Data deposited in or computed by PubChem | |
InChI Key |
VZWXIQHBIQLMPN-UHFFFAOYSA-N | |
Source | PubChem | |
URL | https://pubchem.ncbi.nlm.nih.gov | |
Description | Data deposited in or computed by PubChem | |
Canonical SMILES |
C1CC2=CC=CC=C2OC1 | |
Source | PubChem | |
URL | https://pubchem.ncbi.nlm.nih.gov | |
Description | Data deposited in or computed by PubChem | |
Molecular Formula |
C9H10O | |
Source | PubChem | |
URL | https://pubchem.ncbi.nlm.nih.gov | |
Description | Data deposited in or computed by PubChem | |
DSSTOX Substance ID |
DTXSID10197758 | |
Record name | 2H-1-Benzopyran, 3,4-dihydro- | |
Source | EPA DSSTox | |
URL | https://comptox.epa.gov/dashboard/DTXSID10197758 | |
Description | DSSTox provides a high quality public chemistry resource for supporting improved predictive toxicology. | |
Molecular Weight |
134.17 g/mol | |
Source | PubChem | |
URL | https://pubchem.ncbi.nlm.nih.gov | |
Description | Data deposited in or computed by PubChem | |
CAS No. |
493-08-3 | |
Record name | 3,4-Dihydro-2H-1-benzopyran | |
Source | CAS Common Chemistry | |
URL | https://commonchemistry.cas.org/detail?cas_rn=493-08-3 | |
Description | CAS Common Chemistry is an open community resource for accessing chemical information. Nearly 500,000 chemical substances from CAS REGISTRY cover areas of community interest, including common and frequently regulated chemicals, and those relevant to high school and undergraduate chemistry classes. This chemical information, curated by our expert scientists, is provided in alignment with our mission as a division of the American Chemical Society. | |
Explanation | The data from CAS Common Chemistry is provided under a CC-BY-NC 4.0 license, unless otherwise stated. | |
Record name | Chroman | |
Source | ChemIDplus | |
URL | https://pubchem.ncbi.nlm.nih.gov/substance/?source=chemidplus&sourceid=0000493083 | |
Description | ChemIDplus is a free, web search system that provides access to the structure and nomenclature authority files used for the identification of chemical substances cited in National Library of Medicine (NLM) databases, including the TOXNET system. | |
Record name | 2H-1-Benzopyran, 3,4-dihydro- | |
Source | EPA DSSTox | |
URL | https://comptox.epa.gov/dashboard/DTXSID10197758 | |
Description | DSSTox provides a high quality public chemistry resource for supporting improved predictive toxicology. | |
Record name | 3,4-dihydro-2H-1-benzopyran | |
Source | European Chemicals Agency (ECHA) | |
URL | https://echa.europa.eu/information-on-chemicals | |
Description | The European Chemicals Agency (ECHA) is an agency of the European Union which is the driving force among regulatory authorities in implementing the EU's groundbreaking chemicals legislation for the benefit of human health and the environment as well as for innovation and competitiveness. | |
Explanation | Use of the information, documents and data from the ECHA website is subject to the terms and conditions of this Legal Notice, and subject to other binding limitations provided for under applicable law, the information, documents and data made available on the ECHA website may be reproduced, distributed and/or used, totally or in part, for non-commercial purposes provided that ECHA is acknowledged as the source: "Source: European Chemicals Agency, http://echa.europa.eu/". Such acknowledgement must be included in each copy of the material. ECHA permits and encourages organisations and individuals to create links to the ECHA website under the following cumulative conditions: Links can only be made to webpages that provide a link to the Legal Notice page. | |
Record name | CHROMAN | |
Source | FDA Global Substance Registration System (GSRS) | |
URL | https://gsrs.ncats.nih.gov/ginas/app/beta/substances/EJ8R7LY9PK | |
Description | The FDA Global Substance Registration System (GSRS) enables the efficient and accurate exchange of information on what substances are in regulated products. Instead of relying on names, which vary across regulatory domains, countries, and regions, the GSRS knowledge base makes it possible for substances to be defined by standardized, scientific descriptions. | |
Explanation | Unless otherwise noted, the contents of the FDA website (www.fda.gov), both text and graphics, are not copyrighted. They are in the public domain and may be republished, reprinted and otherwise used freely by anyone without the need to obtain permission from FDA. Credit to the U.S. Food and Drug Administration as the source is appreciated but not required. | |
Synthesis routes and methods I
Procedure details
Synthesis routes and methods II
Procedure details
Synthesis routes and methods III
Procedure details
Synthesis routes and methods IV
Procedure details
Retrosynthesis Analysis
AI-Powered Synthesis Planning: Our tool employs the Template_relevance Pistachio, Template_relevance Bkms_metabolic, Template_relevance Pistachio_ringbreaker, Template_relevance Reaxys, Template_relevance Reaxys_biocatalysis model, leveraging a vast database of chemical reactions to predict feasible synthetic routes.
One-Step Synthesis Focus: Specifically designed for one-step synthesis, it provides concise and direct routes for your target compounds, streamlining the synthesis process.
Accurate Predictions: Utilizing the extensive PISTACHIO, BKMS_METABOLIC, PISTACHIO_RINGBREAKER, REAXYS, REAXYS_BIOCATALYSIS database, our tool offers high-accuracy predictions, reflecting the latest in chemical research and data.
Strategy Settings
Precursor scoring | Relevance Heuristic |
---|---|
Min. plausibility | 0.01 |
Model | Template_relevance |
Template Set | Pistachio/Bkms_metabolic/Pistachio_ringbreaker/Reaxys/Reaxys_biocatalysis |
Top-N result to add to graph | 6 |
Feasible Synthetic Routes
Q1: What is the molecular formula and weight of chromane?
A1: The molecular formula of this compound is C9H10O, and its molecular weight is 134.17 g/mol.
Q2: What synthetic methods are commonly employed to construct this compound derivatives?
A2: Numerous synthetic strategies have been developed for this compound derivatives, including:
- Inverse-electron-demand [4+2] cycloaddition: This method utilizes electron-deficient ortho-quinone methides (o-QMs) and electron-rich dienophiles, such as vinyl ethers [, ]. Notably, this strategy has been successfully employed for the synthesis of 3-alkylchromanes with high stereo- and regioselectivities [].
- Palladium-catalyzed intramolecular allylation: This approach involves the use of indium and indium(III) chloride in conjunction with a palladium catalyst to facilitate the intramolecular allylation of aldehydes with allylic acetates or chlorides, leading to the formation of this compound rings [].
- Indium-mediated intramolecular allylation/allenylation: This strategy exploits the reactivity of organoindium species generated in situ from indium metal to promote intramolecular allylation or allenylation reactions, providing efficient access to this compound derivatives [, ].
- Diels-Alder reaction: This classic cycloaddition reaction can be employed to construct the this compound core, followed by further transformations such as retro-Diels-Alder reaction and oxidative aromatization for the preparation of highly substituted chromanes [].
Q3: What spectroscopic techniques are commonly used to characterize this compound derivatives?
A3: Common spectroscopic techniques employed for the characterization of this compound derivatives include:
- Nuclear Magnetic Resonance (NMR) spectroscopy: NMR spectroscopy, including both 1H and 13C NMR, provides detailed information about the structure, stereochemistry, and conformation of this compound derivatives [, , , ].
- Circular Dichroism (CD) spectroscopy: CD spectroscopy is a valuable tool for investigating the chiroptical properties of chiral this compound derivatives and can be used to determine their absolute configuration [, , , , ].
- Infrared (IR) spectroscopy: IR spectroscopy provides information about the functional groups present in this compound derivatives, such as hydroxyl, carbonyl, and ether groups [].
- Mass spectrometry (MS): MS techniques are used to determine the molecular weight and fragmentation patterns of this compound derivatives, aiding in structural elucidation [, , ].
- X-ray diffraction: This technique allows for the determination of the three-dimensional structure of this compound derivatives in the solid state, providing valuable insights into their conformational preferences and intermolecular interactions [, , , ].
Q4: What are the notable biological activities exhibited by this compound derivatives?
A4: this compound derivatives display a wide range of biological activities, including:
- Antioxidant and anti-inflammatory: Studies have demonstrated that specific this compound and thiothis compound derivatives exhibit potent antioxidant and anti-inflammatory effects by inhibiting reactive oxygen species (ROS) production and the secretion of pro-inflammatory cytokines like interleukin-6 (IL-6) and tumor necrosis factor-α (TNF-α) in macrophages [].
- Antiviral: Certain functionalized chromanols have demonstrated antiviral activity, particularly against poliovirus and herpes simplex virus 1 (HSV-1). The presence of polar groups, such as hydroxyl or aldehyde substituents, has been linked to enhanced activity against these viruses [].
- Antitumor: this compound-containing natural products like berkelic acid and calanolide A have shown promising antitumor and anti-HIV activities, respectively []. Synthetic this compound derivatives have also exhibited cytotoxic activity against various cancer cell lines, including those with multidrug resistance [].
- Potassium channel blockers: Some chromanol compounds, like trans-6-cyano-4-(N-ethylsulfonyl-N-methylamino)-3-hydroxy-2,2-dimethyl- this compound (293B), act as potent blockers of KvLQT1 potassium channels, which play a crucial role in cardiac repolarization [].
Q5: How do the stereochemical features of chromanes influence their biological activity?
A5: The stereochemistry of chiral this compound derivatives plays a crucial role in their biological activity. For instance, in the benzylic hydroxylation of this compound by toluene dioxygenase (TDO) and naphthalene dioxygenase (NDO), stereoselective abstraction of a specific hydrogen atom at the C-4 position occurs, leading to the formation of enantiomerically enriched chroman-4-ols with distinct biological profiles [].
Q6: Are there specific structural modifications of chromanes that are known to enhance or modulate their biological activities?
A6: Yes, the biological activities of chromanes can be significantly influenced by structural modifications:
- Substituents on the this compound ring: The presence and nature of substituents on the this compound ring, particularly at positions 2, 3, and 4, can significantly affect the potency, selectivity, and mechanism of action of these compounds [, , , , ].
- Stereochemistry at C2: The absolute configuration at the C2 position of chiral 2-substituted chromanes has been correlated with their specific optical rotations (SORs), which can influence their interactions with biological targets [].
Q7: What are the future directions for research on this compound derivatives?
A7: Future research directions in the field of this compound chemistry include:
- Exploration of novel synthetic methodologies: The development of new and efficient synthetic methods for accessing diverse and complex this compound derivatives, particularly enantioselective approaches, remains an active area of research [, , ].
- In-depth structure-activity relationship (SAR) studies: Continued investigation into the relationship between the structure of this compound derivatives and their biological activities is essential for optimizing their therapeutic potential [, , , , , ].
- Development of targeted drug delivery systems: Strategies to improve the delivery of this compound-based therapeutics to specific tissues or cells could enhance their efficacy and reduce potential side effects [].
- Investigation of their environmental impact: Assessing the environmental fate and potential ecotoxicological effects of this compound derivatives is crucial for ensuring their responsible use and disposal [].
Q8: What are the potential applications of this compound derivatives in various fields?
A8: The diverse biological activities of this compound derivatives make them promising candidates for applications in various fields, including:
- Medicinal chemistry: Development of novel therapeutic agents for treating a wide range of diseases, such as cancer, inflammation, infectious diseases, and cardiovascular disorders [, , , , , ].
- Agrochemicals: Exploration of their potential as pesticides, herbicides, or fungicides based on their biological activities [].
- Materials science: Utilization of their unique structural features and properties in the development of advanced materials with tailored properties [, ].
体外研究产品的免责声明和信息
请注意,BenchChem 上展示的所有文章和产品信息仅供信息参考。 BenchChem 上可购买的产品专为体外研究设计,这些研究在生物体外进行。体外研究,源自拉丁语 "in glass",涉及在受控实验室环境中使用细胞或组织进行的实验。重要的是要注意,这些产品没有被归类为药物或药品,他们没有得到 FDA 的批准,用于预防、治疗或治愈任何医疗状况、疾病或疾病。我们必须强调,将这些产品以任何形式引入人类或动物的身体都是法律严格禁止的。遵守这些指南对确保研究和实验的法律和道德标准的符合性至关重要。