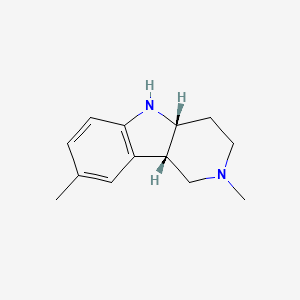
Stobadine
描述
斯托巴丁是一种吡啶吲哚衍生物,以其强大的抗氧化性能而闻名。 它首次合成于 1983 年,此后一直被广泛研究,以期了解其清除活性氧物种和保护组织免受氧化应激的能力 。 斯托巴丁在结构上与吲哚有关,并且已与酚类抗氧化剂 Trolox 进行比较 。
作用机制
斯托巴丁主要通过其抗氧化活性发挥作用。它清除活性氧物种并防止细胞成分受到氧化损伤。斯托巴丁的分子靶标包括活性氧物种和自由基,它通过电子捐赠来中和它们。 这种作用有助于维持细胞完整性并防止氧化应激引起的损伤 。
生化分析
Biochemical Properties
Stobadine plays a significant role in biochemical reactions by interacting with various enzymes, proteins, and other biomolecules. It has been shown to inhibit lipid peroxidation and scavenge free radicals, thereby protecting cellular components from oxidative damage. This compound interacts with enzymes such as superoxide dismutase and catalase, enhancing their activity and contributing to its antioxidant effects .
Cellular Effects
This compound exerts a range of effects on different cell types and cellular processes. It has been demonstrated to protect neuronal cells from oxidative stress-induced apoptosis, thereby preserving cell function and viability. Additionally, this compound influences cell signaling pathways, such as the nuclear factor erythroid 2-related factor 2 (Nrf2) pathway, which regulates the expression of antioxidant genes. This modulation of gene expression helps in maintaining cellular redox balance and preventing oxidative damage .
Molecular Mechanism
The molecular mechanism of this compound involves its ability to directly scavenge reactive oxygen species (ROS) and reactive nitrogen species (RNS). This compound binds to free radicals, neutralizing them and preventing them from causing cellular damage. Furthermore, this compound inhibits the activity of enzymes involved in ROS production, such as NADPH oxidase, thereby reducing oxidative stress. It also upregulates the expression of antioxidant enzymes, enhancing the cell’s defense mechanisms against oxidative damage .
Temporal Effects in Laboratory Settings
In laboratory settings, the effects of this compound have been observed to change over time. This compound is relatively stable and does not undergo significant degradation under standard laboratory conditions. Long-term studies have shown that this compound maintains its antioxidant properties over extended periods, providing sustained protection against oxidative stress. The efficacy of this compound may decrease over time due to potential interactions with other biomolecules in the cellular environment .
Dosage Effects in Animal Models
The effects of this compound vary with different dosages in animal models. At low doses, this compound exhibits potent antioxidant effects without causing any adverse effects. At higher doses, this compound may exhibit toxic effects, including hepatotoxicity and nephrotoxicity. Threshold effects have been observed, where the protective effects of this compound plateau at a certain dosage, beyond which no further benefits are observed .
Metabolic Pathways
This compound is involved in various metabolic pathways, interacting with enzymes and cofactors that regulate its metabolism. It is primarily metabolized in the liver by cytochrome P450 enzymes, resulting in the formation of several metabolites. These metabolites are further conjugated with glucuronic acid or sulfate and excreted via the urine. This compound’s metabolism can influence its bioavailability and efficacy, as well as its potential for drug interactions .
Transport and Distribution
This compound is transported and distributed within cells and tissues through various mechanisms. It can cross cell membranes via passive diffusion and is also transported by specific transporters, such as organic anion-transporting polypeptides (OATPs). Once inside the cell, this compound can accumulate in specific compartments, such as the mitochondria, where it exerts its antioxidant effects. The distribution of this compound within tissues can influence its therapeutic efficacy and potential side effects .
Subcellular Localization
The subcellular localization of this compound is crucial for its activity and function. This compound is primarily localized in the cytoplasm and mitochondria, where it interacts with various biomolecules involved in oxidative stress and antioxidant defense. Post-translational modifications, such as phosphorylation, can influence the targeting and activity of this compound, directing it to specific cellular compartments. The localization of this compound within cells is essential for its ability to protect against oxidative damage and maintain cellular homeostasis .
准备方法
合成路线和反应条件
合成涉及在受控条件下将适当的前体环化以形成吡啶吲哚核心 。反应条件通常包括使用乙醇或甲醇等溶剂和催化剂来促进环化过程。
工业生产方法
对于工业生产,斯托巴丁通常在大型反应器中合成,其中反应条件针对产率和纯度进行了优化。 该过程涉及使用高纯度起始材料和严格的质量控制措施以确保最终产品的一致性 。
化学反应分析
反应类型
斯托巴丁经历了几种类型的化学反应,包括:
氧化: 斯托巴丁可以被氧化以形成各种氧化产物。这种反应通常由过氧化氢等氧化剂促进。
还原: 斯托巴丁的还原反应会导致形成还原衍生物。
常用试剂和条件
氧化剂: 过氧化氢、高锰酸钾。
还原剂: 硼氢化钠、氢化铝锂。
取代试剂: 卤素、烷基化试剂.
形成的主要产物
这些反应形成的主要产物取决于所用试剂和具体条件。 例如,斯托巴丁的氧化会导致形成各种氧化衍生物,而取代反应可以产生一系列取代的吡啶吲哚化合物 。
科学研究应用
斯托巴丁具有广泛的科学研究应用,包括:
化学: 用作模型化合物来研究抗氧化机制和自由基清除。
生物学: 研究其对生物系统中氧化应激的保护作用。
医学: 探索其在涉及氧化应激的疾病(如神经退行性疾病和心血管疾病)中的潜在治疗应用。
工业: 用于开发用于各种工业应用的抗氧化剂配方.
相似化合物的比较
斯托巴丁经常与其他抗氧化剂进行比较,例如:
Trolox: 维生素 E 的水溶性类似物,以其强大的抗氧化性能而闻名。
Cemtirestat: 一种具有抗氧化和醛糖还原酶抑制活性的三嗪吲哚衍生物。
Epalrestat: 一种用于治疗糖尿病并发症的醛糖还原酶抑制剂.
独特性
斯托巴丁的独特性在于它兼具抗氧化剂和神经保护剂的双重作用。 它能够清除多种活性氧物种,并且具有结构稳定性,使其成为研究和治疗应用的宝贵化合物 。
属性
IUPAC Name |
(4aR,9bS)-2,8-dimethyl-1,3,4,4a,5,9b-hexahydropyrido[4,3-b]indole | |
---|---|---|
Source | PubChem | |
URL | https://pubchem.ncbi.nlm.nih.gov | |
Description | Data deposited in or computed by PubChem | |
InChI |
InChI=1S/C13H18N2/c1-9-3-4-12-10(7-9)11-8-15(2)6-5-13(11)14-12/h3-4,7,11,13-14H,5-6,8H2,1-2H3/t11-,13-/m1/s1 | |
Source | PubChem | |
URL | https://pubchem.ncbi.nlm.nih.gov | |
Description | Data deposited in or computed by PubChem | |
InChI Key |
CYJQCYXRNNCURD-DGCLKSJQSA-N | |
Source | PubChem | |
URL | https://pubchem.ncbi.nlm.nih.gov | |
Description | Data deposited in or computed by PubChem | |
Canonical SMILES |
CC1=CC2=C(C=C1)NC3C2CN(CC3)C | |
Source | PubChem | |
URL | https://pubchem.ncbi.nlm.nih.gov | |
Description | Data deposited in or computed by PubChem | |
Isomeric SMILES |
CC1=CC2=C(C=C1)N[C@H]3[C@@H]2CN(CC3)C | |
Source | PubChem | |
URL | https://pubchem.ncbi.nlm.nih.gov | |
Description | Data deposited in or computed by PubChem | |
Molecular Formula |
C13H18N2 | |
Source | PubChem | |
URL | https://pubchem.ncbi.nlm.nih.gov | |
Description | Data deposited in or computed by PubChem | |
DSSTOX Substance ID |
DTXSID201338826 | |
Record name | (4aR,9bS)-2,8-Dimethyl-2,3,4,4a,5,9b-hexahydro-1H-pyrido[4,3-b]indole | |
Source | EPA DSSTox | |
URL | https://comptox.epa.gov/dashboard/DTXSID201338826 | |
Description | DSSTox provides a high quality public chemistry resource for supporting improved predictive toxicology. | |
Molecular Weight |
202.30 g/mol | |
Source | PubChem | |
URL | https://pubchem.ncbi.nlm.nih.gov | |
Description | Data deposited in or computed by PubChem | |
CAS No. |
85202-17-1 | |
Record name | Stobadine | |
Source | ChemIDplus | |
URL | https://pubchem.ncbi.nlm.nih.gov/substance/?source=chemidplus&sourceid=0085202171 | |
Description | ChemIDplus is a free, web search system that provides access to the structure and nomenclature authority files used for the identification of chemical substances cited in National Library of Medicine (NLM) databases, including the TOXNET system. | |
Record name | (4aR,9bS)-2,8-Dimethyl-2,3,4,4a,5,9b-hexahydro-1H-pyrido[4,3-b]indole | |
Source | EPA DSSTox | |
URL | https://comptox.epa.gov/dashboard/DTXSID201338826 | |
Description | DSSTox provides a high quality public chemistry resource for supporting improved predictive toxicology. | |
Record name | STOBADINE | |
Source | FDA Global Substance Registration System (GSRS) | |
URL | https://gsrs.ncats.nih.gov/ginas/app/beta/substances/F9NJ6MT0AP | |
Description | The FDA Global Substance Registration System (GSRS) enables the efficient and accurate exchange of information on what substances are in regulated products. Instead of relying on names, which vary across regulatory domains, countries, and regions, the GSRS knowledge base makes it possible for substances to be defined by standardized, scientific descriptions. | |
Explanation | Unless otherwise noted, the contents of the FDA website (www.fda.gov), both text and graphics, are not copyrighted. They are in the public domain and may be republished, reprinted and otherwise used freely by anyone without the need to obtain permission from FDA. Credit to the U.S. Food and Drug Administration as the source is appreciated but not required. | |
Retrosynthesis Analysis
AI-Powered Synthesis Planning: Our tool employs the Template_relevance Pistachio, Template_relevance Bkms_metabolic, Template_relevance Pistachio_ringbreaker, Template_relevance Reaxys, Template_relevance Reaxys_biocatalysis model, leveraging a vast database of chemical reactions to predict feasible synthetic routes.
One-Step Synthesis Focus: Specifically designed for one-step synthesis, it provides concise and direct routes for your target compounds, streamlining the synthesis process.
Accurate Predictions: Utilizing the extensive PISTACHIO, BKMS_METABOLIC, PISTACHIO_RINGBREAKER, REAXYS, REAXYS_BIOCATALYSIS database, our tool offers high-accuracy predictions, reflecting the latest in chemical research and data.
Strategy Settings
Precursor scoring | Relevance Heuristic |
---|---|
Min. plausibility | 0.01 |
Model | Template_relevance |
Template Set | Pistachio/Bkms_metabolic/Pistachio_ringbreaker/Reaxys/Reaxys_biocatalysis |
Top-N result to add to graph | 6 |
Feasible Synthetic Routes
Q1: What is the primary mechanism of action of Stobadine?
A1: this compound primarily functions as an antioxidant. Its main mechanism of action involves scavenging reactive oxygen species (ROS), including peroxyl radicals and alkoxyl radicals. [, , , , ]
Q2: Does this compound interact directly with any enzymes or receptors?
A2: While this compound's primary action is through ROS scavenging, research suggests interactions with specific enzymes and receptors. Studies have shown that this compound can inhibit voltage-operated ion channels, including sodium, potassium, and calcium channels in rat sensory neurons. [] Additionally, this compound can modulate human neutrophil functionality, particularly by inhibiting superoxide generation and myeloperoxidase release induced by receptor-specific stimuli like fMLP and opsonized zymosan. [] It also interacts with cytochrome P450 in rat liver microsomes, potentially stimulating its oxidase function. []
Q3: What are the downstream effects of this compound's antioxidant activity?
A3: this compound's antioxidant activity leads to several beneficial downstream effects. It can:
- Protect against oxidative damage: This includes protection of lipids, proteins, and DNA in various tissues like the heart, brain, kidneys, and liver. [, , , , ]
- Improve vascular function: this compound can attenuate vascular dysfunction by preserving endothelial function and reducing vasoconstriction. [, ]
- Reduce inflammation: By scavenging ROS, this compound can indirectly reduce the inflammatory response. [, ]
- Modulate cellular signaling: this compound can interfere with signaling pathways involved in inflammation and oxidative stress. [, ]
Q4: What is the molecular formula and weight of this compound?
A4: this compound's molecular formula is C14H18N2 and its molecular weight is 214.31 g/mol. [, ]
Q5: Is there any information available about the spectroscopic data of this compound?
A5: Yes, studies using pulse radiolysis with optical detection showed that one-electron oxidation of this compound generates a radical cation with absorbance maxima at 280 and 445 nm. This radical cation deprotonates to form a nitrogen-centered radical with absorbance maxima at 275, 335, and 410 nm. []
Q6: How do structural modifications of this compound affect its antioxidant activity?
A6: Research suggests that modifications to the this compound structure can significantly impact its antioxidant activity. For instance, dehydrothis compound, a derivative with an aromatized pyridoindole skeleton, exhibits reduced antioxidant activity compared to this compound. [] Furthermore, N-acetylation of the indolic nitrogen in this compound completely abolishes its radical scavenging capacity. [] These findings highlight the importance of the indolic nitrogen center for this compound's antioxidant action. [] A study comparing this compound with its low-basicity derivative, SMe1EC2, found that SMe1EC2 exhibited higher antioxidant activity, potentially due to both improved intrinsic free radical scavenging and better bioavailability. []
Q7: What is known about the stability of this compound in different formulations?
A7: One study examined the bioavailability and pharmacokinetics of two different oral this compound dipalmitate formulations in dogs. Results indicated bioequivalence between the two formulations, suggesting consistent absorption rates despite technological differences. [] Further research is needed to comprehensively understand the stability of this compound under various conditions.
Q8: Are there any specific formulation strategies used to enhance this compound's bioavailability?
A8: While the provided research doesn't explicitly detail formulation strategies for this compound, studies mention the use of dipalmitate salt (DP 1031) for oral administration. [] This suggests that employing prodrug strategies, like using the dipalmitate ester, might be employed to potentially enhance its bioavailability.
Q9: What is known about the absorption, distribution, metabolism, and excretion of this compound?
A9: Studies in rabbits demonstrated that this compound crosses the placental barrier, particularly accumulating in the fetal brain and heart during late gestation. [] Research on rats revealed that this compound is metabolized in the liver, primarily by microsomal enzymes. Two main metabolites have been identified: N-desmethyl this compound and this compound N-oxide, which exists as two stereoisomers. [, ] Further research is necessary to fully elucidate this compound's ADME profile in other species, including humans.
Q10: What evidence supports this compound's in vivo activity and efficacy?
A10: Numerous animal studies highlight this compound's in vivo efficacy in various models:
- Diabetic complications: In diabetic rats, this compound attenuated albuminuria, enzymuria, kidney lipid peroxidation, and matrix collagen cross-linking. [] It also improved NCV deficits and protected against oxidative damage in various tissues. [, , ]
- Ischemia-reperfusion injury: this compound demonstrated protective effects against ischemia-reperfusion injury in various organs, including the heart, brain, kidneys, and intestines. [, , , , ]
- Other models: this compound exhibited protective effects against alloxan-induced hyperglycemia and lipid peroxidation. [, ] It also showed efficacy in reducing gastric mucosal injury and attenuating behavioral changes in stressed mice. [, ]
Q11: What in vitro models have been used to study this compound's effects?
A11: Several in vitro models have been employed to investigate this compound's mechanism of action and potential therapeutic benefits:
- Liposome peroxidation: this compound effectively inhibited lipid peroxidation induced by cumene hydroperoxide and copper ions in liposomal models. [, ]
- Cell-free systems: this compound demonstrated its free radical scavenging capabilities by inhibiting AAPH-induced oxidation in cell-free systems. []
- Isolated cells: Studies utilizing isolated human neutrophils revealed this compound's ability to inhibit superoxide generation and myeloperoxidase release induced by various stimuli. [, , ] Further research using isolated cells and tissues could provide more detailed insights into its cellular and molecular targets.
Q12: Have any clinical trials been conducted with this compound?
A12: While the provided research indicates this compound has progressed to Phase 2 clinical trials [], specific details about these trials are not included.
Q13: What analytical techniques have been used to study this compound?
A13: Various analytical methods have been employed in this compound research:
- Spectrophotometry: Used to monitor conjugated diene formation in lipid peroxidation studies and to analyze this compound's interaction with stable free radicals like DPPH. [, , , ]
- Chromatography: Thin-layer chromatography (TLC) and gas chromatography-mass spectrometry (GC-MS) were utilized for separating and identifying this compound and its metabolites. [, ]
- Mass spectrometry: Employed in conjunction with liquid chromatography (LC-MS/MS) to quantify this compound levels in human plasma. []
- Chemiluminescence: Used to assess the production of reactive oxygen species (ROS) in various biological samples. [, , ]
- Enzyme assays: Various enzyme activities, including superoxide dismutase, glutathione peroxidase, catalase, and N-acetyl-β-D-glucosaminidase, were measured to assess oxidative stress and tissue damage. [, , , , ]
体外研究产品的免责声明和信息
请注意,BenchChem 上展示的所有文章和产品信息仅供信息参考。 BenchChem 上可购买的产品专为体外研究设计,这些研究在生物体外进行。体外研究,源自拉丁语 "in glass",涉及在受控实验室环境中使用细胞或组织进行的实验。重要的是要注意,这些产品没有被归类为药物或药品,他们没有得到 FDA 的批准,用于预防、治疗或治愈任何医疗状况、疾病或疾病。我们必须强调,将这些产品以任何形式引入人类或动物的身体都是法律严格禁止的。遵守这些指南对确保研究和实验的法律和道德标准的符合性至关重要。