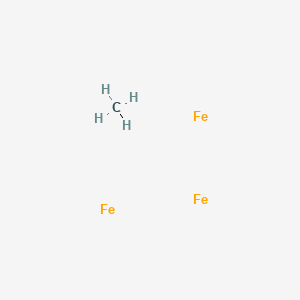
Triiron carbide
概述
描述
Iron carbide is a compound that combines iron and carbon, known for its ceramic-like mechanical behavior and chemical inertness compared to pure iron. It has been used historically in materials such as ancient Damascene steel due to its superior hardness and lightness, and it is found in meteorite iron, which is rich in the carbide phase known as Cohenite["].
合成分析
Reaction Equation:
The synthesis reaction equation for iron carbide (Fe3C) involves the combination of iron (Fe) and carbon (C):Fe+3C→Fe3C
Reaction Conditions:
Iron carbide synthesis can occur under various conditions, including temperature, pressure, and precursor concentration.
Experimental parameters influence the carbon content in iron-carbide nanoparticles["].
Reaction Steps:
While specific steps may vary based on the synthesis method, common steps include:
Precursor Preparation: Prepare iron-containing precursors (e.g., iron salts).
Carbon Source Addition: Introduce a carbon source (e.g., hydrocarbons, carbon monoxide).
Reaction and Heat Treatment: Allow the reaction to proceed, followed by heat treatment to form iron carbide.
Characterization: Analyze the product using techniques like X-ray diffraction and Mössbauer spectroscopy["].
Reaction Mechanism:
The exact mechanism depends on the specific synthesis route (e.g., chemical vapor deposition, sol-gel, or arc discharge).
Generally, carbon atoms diffuse into the iron lattice, leading to the formation of Fe3C["].
分子结构
Atomic Arrangement:
Iron carbide exhibits two basic crystal structures: an orthorhombic form (o) and a hexagonal form (h).
First-principles calculations reveal that the stability of Fe7C3 is comparable to that of cementite (θ−Fe3C).
The orthorhombic phase (o−Fe7C3) is more stable than the hexagonal one, contrary to recent atomistic simulations[“].
A carbon vacancy in the orthorhombic structure suggests possible C deficiency in the lattice.
Bonding Type:
In iron carbide, chemical bonds involve iron (Fe) and carbon (C) atoms.
The primary bonding type is covalent, where electrons are shared between Fe and C.
Metallic bonding also contributes to the overall stability[“].
Geometry:
The orthorhombic phase (o−Fe7C3) has a distinct crystal geometry.
The arrangement of Fe and C atoms forms a complex lattice structure[“].
Electron Cloud Distribution:
Electronic band-structure calculations show that all Fe atoms exhibit high-spin states.
The majority of their 3d states are almost fully occupied.
The electron cloud distribution reflects the metallic character of iron carbide["].
作用机制
Target of Action:
Iron carbide primarily interacts with iron-containing species, especially in the context of carburization processes.
Its primary target is the iron lattice, where carbon atoms are incorporated to form Fe₇C₃["].
Mode of Action:
The carburization process involves the interaction of iron carbide with carbon sources (e.g., methane, carbon monoxide) at elevated temperatures.
The generation of a CHₓ intermediate initiates the overall reaction. This intermediate forms by dissociation of the C–O bond of adsorbed CO[“].
Carbon atoms diffuse into the iron lattice, leading to the formation of Fe₇C₃["].
Result of Action:
The incorporation of carbon into the iron lattice results in the formation of iron carbide (Fe₇C₃).
Fe₇C₃ is a hard, refractory material with applications in various fields, including catalysis, materials science, and even tumor theranostics[“].
Side Effects:
Iron carbide itself is relatively inert and stable.
However, during its synthesis or application, safety precautions are essential due to the high temperatures involved.
Action Environment:
Environmental factors play a role in the carburization process.
High-temperature reduction accelerates the reaction rate and enhances carburization efficiency[“].
The presence of Cl ions can affect the absorption of C atoms, leading to different iron carbide nanostructures[“].
物理属性
State:
Iron carbide exists as a solid at standard temperature and pressure (STP).
It is commonly found in the form of cementite, which is an intermediate transition metal carbide with the formula Fe₃C[“].
Color and Appearance:
The color of iron carbide (cementite) is typically dark gray or black.
It is opaque, meaning it does not allow light to pass through.
The surface appearance can vary, but it tends to be rough due to its crystalline structure.
Density:
The density of iron carbide (Fe₇C₃) is approximately 6.69 g/cm³[“].
This value reflects the mass per unit volume of the solid compound.
Melting Point and Boiling Point:
Iron carbide (Fe₇C₃) has a melting point around 1490°C (approximately 1763 K)[“].
Solubility:
Iron carbide is insoluble in water and most common solvents.
Its stability arises from its strong covalent and metallic bonding.
Hardness:
Iron carbide (cementite) is very hard.
It contributes to the hardness of steel alloys, especially in high-carbon steels.
Electrical Conductivity and Thermal Conductivity:
Iron carbide is a poor conductor of both electricity and heat.
Its metallic lattice structure limits the mobility of electrons.
化学性质
Chemical Reaction Types:
Iron carbide (cementite) participates in various chemical reactions, including:
Carburization: The incorporation of carbon into iron during steel production.
Decomposition: At high temperatures, it decomposes into austenite (low carbon level) and graphite (high carbon level).
Reaction with Oxygen: Forms iron oxide (Fe₂O₃) when exposed to air.
Reaction with Acid: Reacts with strong acids (e.g., hydrochloric acid) to release hydrogen gas.
Reaction with Base: Less reactive with bases due to its covalent nature[“][“].
Reactivity:
Iron carbide is moderately reactive:
It readily reacts with oxygen during carburization.
It is stable in dry environments but corrodes in humid or acidic conditions.
Reactivity depends on temperature and exposure time[“][“].
Redox Property:
Iron carbide is reducing:
During carburization, it reduces iron oxide (Fe₂O₃) to form Fe₃C.
It can act as a reducing agent in certain reactions.
Acidity and Alkalinity:
Iron carbide is neither acidic nor alkaline.
It does not significantly affect pH.
Its neutral behavior is essential for its stability.
Stability:
Iron carbide is thermodynamically unstable:
It eventually converts to austenite and graphite at higher temperatures.
However, it remains stable below the eutectoid temperature (723°C).
Decomposition occurs during prolonged exposure to elevated temperatures[“][“].
Toxicity:
Iron carbide is not inherently toxic.
It is an inert ceramic material.
However, safety precautions are necessary during handling and processing.
生物化学性质
Iron carbide nanoparticles (ICNPs) are nano-intermetallic compounds that consist of iron and carbon[“]. They have been widely applied in energetic and biomedical-related fields[“]. Iron carbide nanoparticles have shown high colloidal stability and good performance in magnetic-dependent diagnosis and therapies such as magnetic resonance imaging (MRI) and magnetic hyperthermia (MH), due to their high magnetization and moderate coercivity[“]. The carbon content protects Iron carbide nanoparticles from oxidation and corrosion (ion release), which prolongs their lifetime and reduces their toxicity in physiological environments.
Cellular Effects: Iron carbide nanoparticles have been proposed as a candidate in nanomedicine, emerging as a promising material for cancer-related diagnosis and treatment[“]. They inherit the unique ferromagnetic and optical properties of iron, while their carbon content provides mechanical strength to the structure[“]. ICNPs have shown high performance in carbon-relevant theranostics. They have great promise in multi-modal imaging or imaging-guided tumor-selective therapy to realize precise diagnoses with mild side effects[“].
Molecular Mechanism: The phases of Fe2C NPs could be controlled by direct phase transformation in the drastic thermally driven procedure[“]. The selective adsorption of Cl ions weakened the bonding between Fe and C atoms, thus interfering with the penetration of C atoms to form lower carbon content Fe5C2 and Fe3C NPs[“]. The kinetic mechanisms were evaluated using density functional theory (DFT) simulations focusing on the bonding energy between Fe–C and Fe–Cl atoms[“].
Time Effect: The rate of production of iron carbides and free carbon depends on the carburizing gas, temperature, and solid reactant properties[“]. An optimum temperature can produce almost pure iron carbide[“]. The reaction mechanism by chemical control proposed in previous studies was also confirmed[“]. High temperature reduction is a useful way to enhance the carburization efficiency, as it can accelerate the reduction reaction rate and carburization reaction rate.
科学研究应用
Iron carbide nanoparticles (ICNPs) are nano-intermetallic compounds composed of iron and carbon. Their unique properties stem from the combination of iron’s magnetic and chemical activity with carbon’s mechanical strength and chemical inertness. These properties make Iron carbide nanoparticles valuable across various fields, including biomedicine, catalysis, and materials science.
Biomedical Applications
Magnetic Resonance Imaging (MRI)
Iron carbide nanoparticles exhibit high magnetization and moderate coercivity, making them excellent candidates for MRI contrast agents. Their colloidal stability ensures accurate imaging, while the carbon content protects them from oxidation and corrosion in physiological environments["].
Magnetic Hyperthermia (MH)
In MH, Iron carbide nanoparticles generate localized heat when exposed to an alternating magnetic field. This property allows targeted hyperthermia treatment for cancer cells, minimizing damage to healthy tissue["].
Magnetic Targeting (MT)
Iron carbide nanoparticles can be functionalized with specific ligands to selectively target tumor cells. MT enhances drug delivery precision, reducing side effects["].
Magnetic Separation (MS)
Iron carbide nanoparticles facilitate efficient separation of biomolecules, cells, or pollutants from complex mixtures. Their magnetic properties enable rapid and precise separation processes["].
Photothermal Therapy (PTT)
When exposed to near-infrared light, Iron carbide nanoparticles convert it into heat, selectively destroying cancer cells. PTT holds promise for non-invasive cancer treatment["].
Photoacoustic Tomography (PAT)
Iron carbide nanoparticles enhance photoacoustic signals, improving the resolution and sensitivity of PAT. This imaging technique combines optical and ultrasound modalities.
Catalysis and Fischer–Tropsch Synthesis
Iron carbides play a crucial role in Fischer–Tropsch synthesis (FTS), a process for converting syngas (a mixture of carbon monoxide and hydrogen) into hydrocarbons. Key applications include:
High-Purity Hägg Carbide (χ-Fe5C2)
Hägg carbide, a specific iron carbide phase, exhibits excellent mechanical strength and chemical inertness. It is a vital component in FTS catalysts, enabling efficient hydrocarbon production["].
Direct Synthesis of Iron Carbide
Recent research focuses on directly synthesizing iron carbide for FTS catalysts. Understanding its structure and reactivity is essential for optimizing catalytic performance.
Functional Nanocomposites
Iron carbide nanoparticles can be embedded within carbon matrices to create functional nanocomposites. By adjusting composition, size, and crystallinity, researchers tailor properties such as magnetism, surface area, and absorption. These nanocomposites find applications in sensors, energy storage, and environmental remediation.
产品比较
Iron carbide,Molybdenum carbide: Similarities and Differences of Compounds
Similarities
Catalytic Activity: Both Iron carbide and Molybdenum carbide are used as catalysts in various industrial processes[“][“]. They are considered alternatives to noble metal catalysts due to their high catalytic activity[“].
Physicochemical Properties: Transition metal carbides, including Iron carbide and Molybdenum carbide, have unique physicochemical properties such as high mechanical hardness, thermal stability, high melting point, resistance to mechanical and corrosive wear, and good thermal and electrical conductivity[“].
Crystal Structure: The crystal structures of transition metal compounds with carbon, including Iron carbide and Molybdenum carbide, can be described as the closest packing of metal atoms with smaller carbon atoms inserted in the interstices[“].
Electronic Structure: Molybdenum carbides are compounds whose electronic structure is similar to the electronic structure of Group IX and X noble metals[“]. This similarity in electronic structure is also found in Iron carbides, making them suitable for similar applications.
Formation of Carbides: The introduction of carbon into the lattice of a transition metal, such as Iron or Molybdenum, leads to an increase in the lattice parameter a0. The metal lattice expands and the metal–metal distance increases to cause d -band contraction (proportional to 1/ a05) upon carbide formation[“].
Resistance to Impurities: Both Iron carbide and Molybdenum carbide can remain active in the presence of impurities in the feedstock, such as N and S impurities[“]. These impurities would not lead to complete catalyst deactivation as they do for noble metal catalysts[“].
Differences
Catalytic Activity: The catalytic activity of Iron carbide and Molybdenum carbide can vary depending on the specific reaction. For example, Iron carbide is often used in Fischer-Tropsch synthesis[“], while Molybdenum carbide shows high catalytic activity in the redox reactions of organic compounds[“].
Crystal Structure: The crystal structures of Iron carbide and Molybdenum carbide are different. Molybdenum carbide is characterized by a hexagonal close-packed crystal structure[“]. On the other hand, the crystal structure of Iron carbide (Fe3C) is orthorhombic.
Carbide Stabilization: Molybdenum is known to act as a carbide stabilizer in cast iron[“]. This property is unique to Molybdenum and is not observed in Iron carbide.
Formation of Carbides: The process conditions for the production of Molybdenum carbide can influence the surface area, morphology, size of crystallites, and the catalytic properties of Molybdenum carbide[“]. This sensitivity to process conditions is not be as pronounced in the formation of Iron carbide.
Mechanical Properties: While both Iron carbide and Molybdenum carbide are hard materials, their mechanical properties can differ. For instance, the hardness, toughness, and wear resistance is vary between these two carbides.
Related Small Molecules
Ferrous sulfate heptahydrate,Iron(III) sulfate hydrate,Iron oxide,Iron,Goethite (Fe(OH)O),Ferric sodium EDTA,Iron dextran
未来方向
Recent advancements in nanotechnologies and material sciences have led to the development of new materials for proton exchange membrane fuel cells. These developments include platinum-based alloys with shape-selected nanostructures, platinum-group-metal-free catalysts such as metal-nitrogen-doped carbon materials, and modifications of the carbon support to control surface properties and ionomer/catalyst interactions. Advanced characterization techniques are contributing to a deeper understanding of catalyst evolution under different conditions. These advancements are critical in reducing costs associated with high platinum cathode loadings, indicating a move towards more cost-effective and efficient clean energy conversion devices[“].
属性
IUPAC Name |
iron;methane | |
---|---|---|
Source | PubChem | |
URL | https://pubchem.ncbi.nlm.nih.gov | |
Description | Data deposited in or computed by PubChem | |
InChI |
InChI=1S/CH4.3Fe/h1H4;;; | |
Source | PubChem | |
URL | https://pubchem.ncbi.nlm.nih.gov | |
Description | Data deposited in or computed by PubChem | |
InChI Key |
KSOKAHYVTMZFBJ-UHFFFAOYSA-N | |
Source | PubChem | |
URL | https://pubchem.ncbi.nlm.nih.gov | |
Description | Data deposited in or computed by PubChem | |
Canonical SMILES |
C.[Fe].[Fe].[Fe] | |
Source | PubChem | |
URL | https://pubchem.ncbi.nlm.nih.gov | |
Description | Data deposited in or computed by PubChem | |
Molecular Formula |
CH4Fe3 | |
Source | PubChem | |
URL | https://pubchem.ncbi.nlm.nih.gov | |
Description | Data deposited in or computed by PubChem | |
Molecular Weight |
183.58 g/mol | |
Source | PubChem | |
URL | https://pubchem.ncbi.nlm.nih.gov | |
Description | Data deposited in or computed by PubChem | |
Physical Description |
Other Solid; Pellets or Large Crystals, Grey cubic powder; [Alfa Aesar MSDS] | |
Record name | Iron carbide (Fe3C) | |
Source | EPA Chemicals under the TSCA | |
URL | https://www.epa.gov/chemicals-under-tsca | |
Description | EPA Chemicals under the Toxic Substances Control Act (TSCA) collection contains information on chemicals and their regulations under TSCA, including non-confidential content from the TSCA Chemical Substance Inventory and Chemical Data Reporting. | |
Record name | Iron carbide | |
Source | Haz-Map, Information on Hazardous Chemicals and Occupational Diseases | |
URL | https://haz-map.com/Agents/14931 | |
Description | Haz-Map® is an occupational health database designed for health and safety professionals and for consumers seeking information about the adverse effects of workplace exposures to chemical and biological agents. | |
Explanation | Copyright (c) 2022 Haz-Map(R). All rights reserved. Unless otherwise indicated, all materials from Haz-Map are copyrighted by Haz-Map(R). No part of these materials, either text or image may be used for any purpose other than for personal use. Therefore, reproduction, modification, storage in a retrieval system or retransmission, in any form or by any means, electronic, mechanical or otherwise, for reasons other than personal use, is strictly prohibited without prior written permission. | |
CAS No. |
12011-67-5 | |
Record name | Iron carbide (Fe3C) | |
Source | ChemIDplus | |
URL | https://pubchem.ncbi.nlm.nih.gov/substance/?source=chemidplus&sourceid=0012011675 | |
Description | ChemIDplus is a free, web search system that provides access to the structure and nomenclature authority files used for the identification of chemical substances cited in National Library of Medicine (NLM) databases, including the TOXNET system. | |
Record name | Iron carbide (Fe3C) | |
Source | EPA Chemicals under the TSCA | |
URL | https://www.epa.gov/chemicals-under-tsca | |
Description | EPA Chemicals under the Toxic Substances Control Act (TSCA) collection contains information on chemicals and their regulations under TSCA, including non-confidential content from the TSCA Chemical Substance Inventory and Chemical Data Reporting. | |
Record name | Triiron carbide | |
Source | European Chemicals Agency (ECHA) | |
URL | https://echa.europa.eu/substance-information/-/substanceinfo/100.031.411 | |
Description | The European Chemicals Agency (ECHA) is an agency of the European Union which is the driving force among regulatory authorities in implementing the EU's groundbreaking chemicals legislation for the benefit of human health and the environment as well as for innovation and competitiveness. | |
Explanation | Use of the information, documents and data from the ECHA website is subject to the terms and conditions of this Legal Notice, and subject to other binding limitations provided for under applicable law, the information, documents and data made available on the ECHA website may be reproduced, distributed and/or used, totally or in part, for non-commercial purposes provided that ECHA is acknowledged as the source: "Source: European Chemicals Agency, http://echa.europa.eu/". Such acknowledgement must be included in each copy of the material. ECHA permits and encourages organisations and individuals to create links to the ECHA website under the following cumulative conditions: Links can only be made to webpages that provide a link to the Legal Notice page. | |
Retrosynthesis Analysis
AI-Powered Synthesis Planning: Our tool employs the Template_relevance Pistachio, Template_relevance Bkms_metabolic, Template_relevance Pistachio_ringbreaker, Template_relevance Reaxys, Template_relevance Reaxys_biocatalysis model, leveraging a vast database of chemical reactions to predict feasible synthetic routes.
One-Step Synthesis Focus: Specifically designed for one-step synthesis, it provides concise and direct routes for your target compounds, streamlining the synthesis process.
Accurate Predictions: Utilizing the extensive PISTACHIO, BKMS_METABOLIC, PISTACHIO_RINGBREAKER, REAXYS, REAXYS_BIOCATALYSIS database, our tool offers high-accuracy predictions, reflecting the latest in chemical research and data.
Strategy Settings
Precursor scoring | Relevance Heuristic |
---|---|
Min. plausibility | 0.01 |
Model | Template_relevance |
Template Set | Pistachio/Bkms_metabolic/Pistachio_ringbreaker/Reaxys/Reaxys_biocatalysis |
Top-N result to add to graph | 6 |
Feasible Synthetic Routes
体外研究产品的免责声明和信息
请注意,BenchChem 上展示的所有文章和产品信息仅供信息参考。 BenchChem 上可购买的产品专为体外研究设计,这些研究在生物体外进行。体外研究,源自拉丁语 "in glass",涉及在受控实验室环境中使用细胞或组织进行的实验。重要的是要注意,这些产品没有被归类为药物或药品,他们没有得到 FDA 的批准,用于预防、治疗或治愈任何医疗状况、疾病或疾病。我们必须强调,将这些产品以任何形式引入人类或动物的身体都是法律严格禁止的。遵守这些指南对确保研究和实验的法律和道德标准的符合性至关重要。