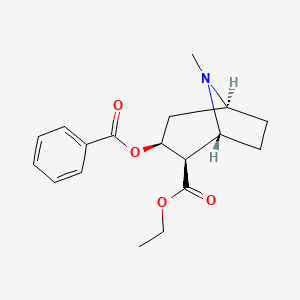
可卡乙烯
描述
Cocaethylene, also known as ethylbenzoylecgonine, is the ethyl ester of benzoylecgonine . It is structurally similar to cocaine, which is the methyl ester of benzoylecgonine . Cocaethylene is formed by the liver when cocaine and ethanol coexist in the blood . It is largely considered a recreational drug in and of itself, with stimulant, euphoriant, anorectic, sympathomimetic, and local anesthetic properties .
Synthesis Analysis
Cocaethylene is the byproduct of concurrent consumption of alcohol and cocaine as metabolized by the liver . Normally, metabolism of cocaine produces two primarily biologically inactive metabolites — benzoylecgonine and ecgonine methyl ester . If ethanol is present during the metabolism of cocaine, a portion of the cocaine undergoes transesterification with ethanol, rather than undergoing hydrolysis with water, which results in the production of cocaethylene .
Molecular Structure Analysis
Cocaethylene has a molecular formula of C18H23NO4 and a molar mass of 317.385 g·mol −1 . It is the ethyl ester of benzoylecgonine .
Chemical Reactions Analysis
The hepatic enzyme carboxylesterase is an important part of cocaine’s metabolism because it acts as a catalyst for the hydrolysis of cocaine in the liver, which produces these inactive metabolites . If ethanol is present during the metabolism of cocaine, a portion of the cocaine undergoes transesterification with ethanol, rather than undergoing hydrolysis with water, which results in the production of cocaethylene .
Physical And Chemical Properties Analysis
Cocaethylene and cocaine are equi-effective at blocking the reuptake of dopamine at receptor sites, thus reinforcing the stimulant effects of the neurotransmitter . The plasma elimination half-life of Cocaethylene is 2 h compared with 1 h for cocaine .
科学研究应用
Pharmacokinetics and Metabolism
Cocaethylene (CE) is formed in the body when ethanol and cocaine are co-ingested. It has a plasma elimination half-life of approximately 2 hours , which is longer than cocaine’s half-life of about 1 hour . Understanding the pharmacokinetics of CE is crucial for forensic toxicology, as it helps in determining the timing and extent of cocaine and alcohol use.
Forensic Toxicology
CE is a significant metabolite in forensic cases involving the co-ingestion of cocaine and alcohol. It’s often encountered in routine casework and can be more toxic to the heart and liver compared to cocaine . Forensic toxicologists utilize the presence of CE to interpret toxicological results in relation to acute intoxication and overdose deaths.
Neuropharmacology
Both CE and cocaine are equi-effective at blocking the reuptake of dopamine at receptor sites, reinforcing the stimulant effects of the neurotransmitter . This property makes CE an important subject of study in neuropharmacology, particularly concerning its effects on the central nervous system and its addictive potential.
Cardiotoxicity Studies
CE is considered more toxic to the heart than cocaine. Research into CE’s cardiotoxic effects is vital for understanding the risks associated with recreational drug use, especially in scenarios where individuals consume both cocaine and alcohol .
Hepatotoxicity Research
Given that CE has a higher toxicity to the liver, studies focusing on hepatotoxicity are essential. These research applications aim to understand the long-term effects of CE on liver function and its potential to cause liver damage .
Behavioral Sciences
The behavioral effects of CE, due to its impact on dopamine reuptake, are a subject of interest in behavioral sciences. Research in this field explores how CE influences behavior, addiction, and the psychological effects of substance abuse .
Analytical Chemistry
Developing methods for the detection and quantification of CE is a key application in analytical chemistry. Accurate measurement of CE levels is necessary for both clinical and forensic purposes, aiding in the assessment of drug abuse patterns .
Biomarker for Substance Abuse
CE serves as a biomarker for the co-consumption of cocaine with alcohol. Its presence in biological samples indicates the use of both substances, which is valuable information in clinical diagnostics and treatment of substance abuse disorders .
作用机制
Cocaethylene is a psychoactive compound formed in the liver when cocaine and ethanol coexist in the blood . It shares structural similarities with cocaine and has been associated with enhanced subjective euphoria, increased heart rate, and increased plasma cocaine concentration .
Target of Action
Cocaethylene primarily targets the monoamine neurotransmitters serotonin, norepinephrine, and dopamine in the brain . These neurotransmitters play crucial roles in mood regulation, arousal, and reward systems.
Mode of Action
Cocaethylene increases the levels of serotonergic, noradrenergic, and dopaminergic neurotransmission in the brain by inhibiting the action of the serotonin transporter, norepinephrine transporter, and dopamine transporter . This inhibition results in an increased concentration of these neurotransmitters in the synaptic cleft, leading to enhanced neurotransmission.
Biochemical Pathways
Cocaethylene is the byproduct of concurrent consumption of alcohol and cocaine, metabolized by the liver . Normally, metabolism of cocaine produces two primarily biologically inactive metabolites — benzoylecgonine and ecgonine methyl ester. If ethanol is present during the metabolism of cocaine, a portion of the cocaine undergoes transesterification with ethanol, rather than undergoing hydrolysis with water, which results in the production of cocaethylene .
Pharmacokinetics
It is known that cocaethylene has a longer duration of action than cocaine . The presence of ethanol during the metabolism of cocaine can also increase the peak concentration of cocaine by roughly 20% .
Result of Action
Cocaethylene is largely considered a recreational drug in and of itself, with stimulant, euphoriant, anorectic, sympathomimetic, and local anesthetic properties . In most users, cocaethylene produces euphoria and has a longer duration of action than cocaine . Some studies suggest that consuming alcohol in combination with cocaine may be more cardiotoxic than cocaine and "it also carries an 18 to 25 fold increase over cocaine alone in risk of immediate death" .
Action Environment
The action, efficacy, and stability of cocaethylene can be influenced by various environmental factors. For instance, misuse of cocaine can disrupt the body’s thermoregulation, which can lead to fatalities from hyperthermia, especially when the drug is misused in a hot climate and at higher environmental temperature .
安全和危害
Cocaethylene is considered more toxic to the heart and liver compared with the parent drug cocaine . Some studies suggest that consuming alcohol in combination with cocaine may be more cardiotoxic than cocaine and "it also carries an 18 to 25 fold increase over cocaine alone in risk of immediate death" . According to its safety data sheet, it is highly flammable and causes serious eye irritation .
未来方向
While Cocaethylene has been known to science for decades, it has not been extensively studied and even its metabolic pathways are not entirely elucidated . Greater awareness of Cocaethylene is needed for clinicians, and those who use cocaine should also be aware of the potential for polysubstance use of cocaine and ethanol to produce a potentially potent and long-lasting psychoactive metabolite .
属性
IUPAC Name |
ethyl (1R,2R,3S,5S)-3-benzoyloxy-8-methyl-8-azabicyclo[3.2.1]octane-2-carboxylate | |
---|---|---|
Source | PubChem | |
URL | https://pubchem.ncbi.nlm.nih.gov | |
Description | Data deposited in or computed by PubChem | |
InChI |
InChI=1S/C18H23NO4/c1-3-22-18(21)16-14-10-9-13(19(14)2)11-15(16)23-17(20)12-7-5-4-6-8-12/h4-8,13-16H,3,9-11H2,1-2H3/t13-,14+,15-,16+/m0/s1 | |
Source | PubChem | |
URL | https://pubchem.ncbi.nlm.nih.gov | |
Description | Data deposited in or computed by PubChem | |
InChI Key |
NMPOSNRHZIWLLL-XUWVNRHRSA-N | |
Source | PubChem | |
URL | https://pubchem.ncbi.nlm.nih.gov | |
Description | Data deposited in or computed by PubChem | |
Canonical SMILES |
CCOC(=O)C1C2CCC(N2C)CC1OC(=O)C3=CC=CC=C3 | |
Source | PubChem | |
URL | https://pubchem.ncbi.nlm.nih.gov | |
Description | Data deposited in or computed by PubChem | |
Isomeric SMILES |
CCOC(=O)[C@@H]1[C@H]2CC[C@H](N2C)C[C@@H]1OC(=O)C3=CC=CC=C3 | |
Source | PubChem | |
URL | https://pubchem.ncbi.nlm.nih.gov | |
Description | Data deposited in or computed by PubChem | |
Molecular Formula |
C18H23NO4 | |
Source | PubChem | |
URL | https://pubchem.ncbi.nlm.nih.gov | |
Description | Data deposited in or computed by PubChem | |
DSSTOX Substance ID |
DTXSID20873213 | |
Record name | Cocaethylene | |
Source | EPA DSSTox | |
URL | https://comptox.epa.gov/dashboard/DTXSID20873213 | |
Description | DSSTox provides a high quality public chemistry resource for supporting improved predictive toxicology. | |
Molecular Weight |
317.4 g/mol | |
Source | PubChem | |
URL | https://pubchem.ncbi.nlm.nih.gov | |
Description | Data deposited in or computed by PubChem | |
Product Name |
Cocaethylene | |
CAS RN |
529-38-4 | |
Record name | Cocaethylene | |
Source | CAS Common Chemistry | |
URL | https://commonchemistry.cas.org/detail?cas_rn=529-38-4 | |
Description | CAS Common Chemistry is an open community resource for accessing chemical information. Nearly 500,000 chemical substances from CAS REGISTRY cover areas of community interest, including common and frequently regulated chemicals, and those relevant to high school and undergraduate chemistry classes. This chemical information, curated by our expert scientists, is provided in alignment with our mission as a division of the American Chemical Society. | |
Explanation | The data from CAS Common Chemistry is provided under a CC-BY-NC 4.0 license, unless otherwise stated. | |
Record name | Cocaethylene | |
Source | ChemIDplus | |
URL | https://pubchem.ncbi.nlm.nih.gov/substance/?source=chemidplus&sourceid=0000529384 | |
Description | ChemIDplus is a free, web search system that provides access to the structure and nomenclature authority files used for the identification of chemical substances cited in National Library of Medicine (NLM) databases, including the TOXNET system. | |
Record name | Cocaethylene | |
Source | EPA DSSTox | |
URL | https://comptox.epa.gov/dashboard/DTXSID20873213 | |
Description | DSSTox provides a high quality public chemistry resource for supporting improved predictive toxicology. | |
Record name | 8-Azabicyclo(3.2.1)octane-2-carboxylic acid, 3-(benzoyloxy)-8-methyl-, ethyl ester, (1R-(exo,exo))- | |
Source | European Chemicals Agency (ECHA) | |
URL | https://echa.europa.eu/information-on-chemicals | |
Description | The European Chemicals Agency (ECHA) is an agency of the European Union which is the driving force among regulatory authorities in implementing the EU's groundbreaking chemicals legislation for the benefit of human health and the environment as well as for innovation and competitiveness. | |
Explanation | Use of the information, documents and data from the ECHA website is subject to the terms and conditions of this Legal Notice, and subject to other binding limitations provided for under applicable law, the information, documents and data made available on the ECHA website may be reproduced, distributed and/or used, totally or in part, for non-commercial purposes provided that ECHA is acknowledged as the source: "Source: European Chemicals Agency, http://echa.europa.eu/". Such acknowledgement must be included in each copy of the material. ECHA permits and encourages organisations and individuals to create links to the ECHA website under the following cumulative conditions: Links can only be made to webpages that provide a link to the Legal Notice page. | |
Record name | COCAETHYLENE | |
Source | FDA Global Substance Registration System (GSRS) | |
URL | https://gsrs.ncats.nih.gov/ginas/app/beta/substances/FJO3071W5Y | |
Description | The FDA Global Substance Registration System (GSRS) enables the efficient and accurate exchange of information on what substances are in regulated products. Instead of relying on names, which vary across regulatory domains, countries, and regions, the GSRS knowledge base makes it possible for substances to be defined by standardized, scientific descriptions. | |
Explanation | Unless otherwise noted, the contents of the FDA website (www.fda.gov), both text and graphics, are not copyrighted. They are in the public domain and may be republished, reprinted and otherwise used freely by anyone without the need to obtain permission from FDA. Credit to the U.S. Food and Drug Administration as the source is appreciated but not required. | |
Retrosynthesis Analysis
AI-Powered Synthesis Planning: Our tool employs the Template_relevance Pistachio, Template_relevance Bkms_metabolic, Template_relevance Pistachio_ringbreaker, Template_relevance Reaxys, Template_relevance Reaxys_biocatalysis model, leveraging a vast database of chemical reactions to predict feasible synthetic routes.
One-Step Synthesis Focus: Specifically designed for one-step synthesis, it provides concise and direct routes for your target compounds, streamlining the synthesis process.
Accurate Predictions: Utilizing the extensive PISTACHIO, BKMS_METABOLIC, PISTACHIO_RINGBREAKER, REAXYS, REAXYS_BIOCATALYSIS database, our tool offers high-accuracy predictions, reflecting the latest in chemical research and data.
Strategy Settings
Precursor scoring | Relevance Heuristic |
---|---|
Min. plausibility | 0.01 |
Model | Template_relevance |
Template Set | Pistachio/Bkms_metabolic/Pistachio_ringbreaker/Reaxys/Reaxys_biocatalysis |
Top-N result to add to graph | 6 |
Feasible Synthetic Routes
体外研究产品的免责声明和信息
请注意,BenchChem 上展示的所有文章和产品信息仅供信息参考。 BenchChem 上可购买的产品专为体外研究设计,这些研究在生物体外进行。体外研究,源自拉丁语 "in glass",涉及在受控实验室环境中使用细胞或组织进行的实验。重要的是要注意,这些产品没有被归类为药物或药品,他们没有得到 FDA 的批准,用于预防、治疗或治愈任何医疗状况、疾病或疾病。我们必须强调,将这些产品以任何形式引入人类或动物的身体都是法律严格禁止的。遵守这些指南对确保研究和实验的法律和道德标准的符合性至关重要。