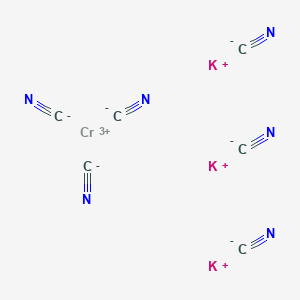
Hexacyanochromate
描述
Hexacyanochromate, also known as this compound, is a useful research compound. Its molecular formula is C6CrK3N6 and its molecular weight is 325.4 g/mol. The purity is usually 95%.
BenchChem offers high-quality this compound suitable for many research applications. Different packaging options are available to accommodate customers' requirements. Please inquire for more information about this compound including the price, delivery time, and more detailed information at info@benchchem.com.
生化分析
Biochemical Properties
Hexacyanochromate plays a significant role in biochemical reactions, particularly as a paramagnetic anion probe for active sites of enzymes. It interacts with enzymes such as ribonuclease A, where it binds to the active site and selectively broadens the proton signals of specific histidine residues . This interaction is useful for mapping the active sites of enzymes for anionic substrates. Additionally, this compound has been studied for its magnetocaloric properties in Prussian blue analogs, indicating its potential in biochemical applications involving magnetic entropy changes .
Cellular Effects
This compound influences various cellular processes, including cell signaling pathways, gene expression, and cellular metabolism. It has been observed to affect the magnetocaloric properties of molecule-based materials, which can impact cellular functions related to temperature regulation and magnetic field responses . The compound’s paramagnetic nature allows it to interact with cellular components, potentially altering cell signaling pathways and gene expression patterns.
Molecular Mechanism
At the molecular level, this compound exerts its effects through binding interactions with biomolecules. It binds to the active sites of enzymes, such as ribonuclease A, and induces changes in the proton signals of specific residues . This binding interaction can lead to enzyme inhibition or activation, depending on the nature of the enzyme and the specific binding site. Additionally, this compound’s paramagnetic properties enable it to influence magnetic field responses at the molecular level .
Temporal Effects in Laboratory Settings
In laboratory settings, the effects of this compound can change over time due to factors such as stability and degradation. Studies have shown that the compound remains stable under certain conditions, but its effects on cellular function can vary depending on the duration of exposure and environmental factors . Long-term effects on cellular function have been observed in both in vitro and in vivo studies, indicating the importance of monitoring the compound’s stability and degradation over time.
Dosage Effects in Animal Models
The effects of this compound vary with different dosages in animal models. At lower dosages, the compound may exhibit beneficial effects on cellular function and metabolism. At higher dosages, toxic or adverse effects can occur, including potential damage to cellular components and disruption of metabolic pathways . Threshold effects have been observed, highlighting the importance of determining the optimal dosage for specific applications.
Metabolic Pathways
This compound is involved in various metabolic pathways, interacting with enzymes and cofactors that regulate metabolic flux and metabolite levels. The compound’s interactions with enzymes such as ribonuclease A can influence the overall metabolic activity within cells . Additionally, this compound’s paramagnetic properties may affect the regulation of metabolic pathways involving magnetic field responses .
Transport and Distribution
Within cells and tissues, this compound is transported and distributed through interactions with transporters and binding proteins. These interactions can influence the compound’s localization and accumulation in specific cellular compartments . The transport and distribution of this compound are critical for its biochemical activity and overall effectiveness in various applications.
Subcellular Localization
This compound’s subcellular localization plays a crucial role in its activity and function. The compound can be directed to specific compartments or organelles through targeting signals or post-translational modifications . Its localization within subcellular structures can influence its interactions with biomolecules and its overall biochemical effects.
属性
IUPAC Name |
tripotassium;chromium(3+);hexacyanide | |
---|---|---|
Source | PubChem | |
URL | https://pubchem.ncbi.nlm.nih.gov | |
Description | Data deposited in or computed by PubChem | |
InChI |
InChI=1S/6CN.Cr.3K/c6*1-2;;;;/q6*-1;+3;3*+1 | |
Source | PubChem | |
URL | https://pubchem.ncbi.nlm.nih.gov | |
Description | Data deposited in or computed by PubChem | |
InChI Key |
XYBUIQUQPGBKAR-UHFFFAOYSA-N | |
Source | PubChem | |
URL | https://pubchem.ncbi.nlm.nih.gov | |
Description | Data deposited in or computed by PubChem | |
Canonical SMILES |
[C-]#N.[C-]#N.[C-]#N.[C-]#N.[C-]#N.[C-]#N.[K+].[K+].[K+].[Cr+3] | |
Source | PubChem | |
URL | https://pubchem.ncbi.nlm.nih.gov | |
Description | Data deposited in or computed by PubChem | |
Molecular Formula |
C6CrK3N6 | |
Source | PubChem | |
URL | https://pubchem.ncbi.nlm.nih.gov | |
Description | Data deposited in or computed by PubChem | |
DSSTOX Substance ID |
DTXSID10929218 | |
Record name | (OC-6-11)-Tripotassium hexakis(cyano-C)chromate(3-) | |
Source | EPA DSSTox | |
URL | https://comptox.epa.gov/dashboard/DTXSID10929218 | |
Description | DSSTox provides a high quality public chemistry resource for supporting improved predictive toxicology. | |
Molecular Weight |
325.40 g/mol | |
Source | PubChem | |
URL | https://pubchem.ncbi.nlm.nih.gov | |
Description | Data deposited in or computed by PubChem | |
CAS No. |
13601-11-1 | |
Record name | Hexacyanochromate | |
Source | ChemIDplus | |
URL | https://pubchem.ncbi.nlm.nih.gov/substance/?source=chemidplus&sourceid=0013601111 | |
Description | ChemIDplus is a free, web search system that provides access to the structure and nomenclature authority files used for the identification of chemical substances cited in National Library of Medicine (NLM) databases, including the TOXNET system. | |
Record name | (OC-6-11)-Tripotassium hexakis(cyano-C)chromate(3-) | |
Source | EPA DSSTox | |
URL | https://comptox.epa.gov/dashboard/DTXSID10929218 | |
Description | DSSTox provides a high quality public chemistry resource for supporting improved predictive toxicology. | |
Record name | Tripotassium hexa(cyano-C)chromate(3-) | |
Source | European Chemicals Agency (ECHA) | |
URL | https://echa.europa.eu/substance-information/-/substanceinfo/100.033.694 | |
Description | The European Chemicals Agency (ECHA) is an agency of the European Union which is the driving force among regulatory authorities in implementing the EU's groundbreaking chemicals legislation for the benefit of human health and the environment as well as for innovation and competitiveness. | |
Explanation | Use of the information, documents and data from the ECHA website is subject to the terms and conditions of this Legal Notice, and subject to other binding limitations provided for under applicable law, the information, documents and data made available on the ECHA website may be reproduced, distributed and/or used, totally or in part, for non-commercial purposes provided that ECHA is acknowledged as the source: "Source: European Chemicals Agency, http://echa.europa.eu/". Such acknowledgement must be included in each copy of the material. ECHA permits and encourages organisations and individuals to create links to the ECHA website under the following cumulative conditions: Links can only be made to webpages that provide a link to the Legal Notice page. | |
Retrosynthesis Analysis
AI-Powered Synthesis Planning: Our tool employs the Template_relevance Pistachio, Template_relevance Bkms_metabolic, Template_relevance Pistachio_ringbreaker, Template_relevance Reaxys, Template_relevance Reaxys_biocatalysis model, leveraging a vast database of chemical reactions to predict feasible synthetic routes.
One-Step Synthesis Focus: Specifically designed for one-step synthesis, it provides concise and direct routes for your target compounds, streamlining the synthesis process.
Accurate Predictions: Utilizing the extensive PISTACHIO, BKMS_METABOLIC, PISTACHIO_RINGBREAKER, REAXYS, REAXYS_BIOCATALYSIS database, our tool offers high-accuracy predictions, reflecting the latest in chemical research and data.
Strategy Settings
Precursor scoring | Relevance Heuristic |
---|---|
Min. plausibility | 0.01 |
Model | Template_relevance |
Template Set | Pistachio/Bkms_metabolic/Pistachio_ringbreaker/Reaxys/Reaxys_biocatalysis |
Top-N result to add to graph | 6 |
Feasible Synthetic Routes
Q1: What is the molecular formula of hexacyanochromate(III) ion?
A1: The molecular formula of the this compound(III) ion is [Cr(CN)6]3-.
Q2: What are the characteristic spectroscopic features of this compound(III) compounds?
A2: this compound(III) compounds exhibit distinct spectroscopic features, particularly in infrared (IR) spectroscopy. The cyanide (CN) ligands exhibit characteristic stretching frequencies (νCN) that can be used to identify the presence of these compounds and gain insights into their bonding and structure. [, , ]
Q3: How does the potassium this compound(III) crystal structure differ at low temperatures?
A3: At 4.2 K, potassium this compound(III) adopts a triclinic crystal structure (space group P1), which arises from symmetry-determined translations of the potassium (K+) and this compound(III) ions from its higher temperature face-centered cubic structure. This low-temperature structure also exhibits twinning and stacking disorder. []
Q4: Are there stability concerns with transition metal this compound(III) compounds?
A4: Yes, single metal salts of transition metal this compound(III) compounds, with the general formula T3[Cr(CN)6]2·yH2O (T = Mn2+, Fe2+, Co2+), exhibit low stability. This instability is attributed to the potential for cyanide ligand flipping, transitioning from a T-N≡C-Cr-C≡N-T configuration to Cr-N≡C-T-C≡N-Cr. []
Q5: Can the stability of these compounds be improved?
A5: Partially. Incorporating a second metal ion that does not readily form stable hexacyano complexes, such as nickel (Ni), copper (Cu), zinc (Zn), or cadmium (Cd), can enhance their stability. [] Additionally, compact analogs like TCs[Cr(CN)6] show greater stability over time. []
Q6: How does pressure impact the structure and magnetic properties of nickel this compound?
A6: Applying pressure to nickel this compound, a Prussian blue analog, induces structural changes, including the isomerization of bridging cyanide ligands. This isomerization alters the local ligand field and introduces inhomogeneities in the magnetic anisotropy. These changes hinder magnetic domain movement, leading to a decrease in low-field magnetic susceptibility. []
Q7: How does the incorporation of carbon nanotubes affect cobalt tetracyanonickelate this compound?
A7: Decorating cobalt tetracyanonickelate this compound with carbon nanotubes (CNTs) leads to a blue shift in the optical band gap, as observed in the transmittance and reflectance spectra. []
Q8: What unique application does the this compound(III) ion offer in enzyme studies?
A8: The this compound(III) ion serves as a paramagnetic anion probe for investigating the active sites of enzymes that interact with anionic substrates. Its paramagnetic nature allows for studies using nuclear magnetic resonance (NMR) spectroscopy. For instance, in ribonuclease A, the ion binds to the active site, causing selective broadening of specific proton signals in the NMR spectrum. This broadening provides insights into the enzyme's active site structure and interactions. []
Q9: How do ribonucleotides interact with certain metal hexacyanochromates(III)?
A9: Ribonucleotides, such as 5′-AMP, 5′-GMP, 5′-CMP, and 5′-UMP, demonstrate notable adsorption onto cobalt(II) and cadmium(II) this compound(III) compounds. This adsorption is maximized under neutral pH conditions, suggesting potential applications in areas like chemical evolution studies. []
Q10: How is this compound(III) employed in synthesizing magnetic thin films?
A10: this compound(III) plays a crucial role in the ion-exchange synthesis of magnetic thin films based on metal(II) this compound(III) compounds. These films, with the general formula MII1.5[CrIII(CN)6].ZH2O (M = Co, Ni, Cu), are prepared on transparent Nafion membranes and exhibit interesting magneto-optical properties. []
Q11: Can you elaborate on the energy transfer processes observed in crystals containing [M(bpy)3]2+ (M = Ru, Os) and this compound(III)?
A11: In crystals of double-complex salts like [M(bpy)3]2[Cr(CN)6]Cl.8H2O (M = Ru2+, Os2+; bpy = 2,2'-bipyridine), energy transfer occurs from the photoexcited [M(bpy)3]2+ to the [Cr(CN)6]3-. This transfer is attributed to intermolecular energy transfer from the excited state of the ruthenium or osmium complex to the ligand-field excited state of the this compound(III) unit. This phenomenon has been investigated through luminescence studies and provides insights into the photophysical properties of these systems. [, ]
Q12: Can this compound(III) be used in the development of low-potential battery materials?
A12: Research has explored the potential of manganese this compound as a low-potential material for sodium-ion batteries. This material exhibits reversible sodium insertion at a low potential while maintaining its structural integrity. [, ]
Q13: How does the particle size of iron(II) this compound(III) affect its properties?
A13: Decreasing the particle size of iron(II) this compound(III) nanoparticles leads to a faster rate of linkage isomerization. Kinetic analyses at different temperatures reveal that the activation energy of this isomerization process is inversely related to particle size. []
Q14: How has computational chemistry contributed to understanding spin crossover in cesium iron this compound?
A14: Computational studies, particularly periodic density functional theory (DFT) calculations, have played a crucial role in elucidating the spin crossover behavior observed in cesium iron this compound. These calculations suggest that the spin transition is primarily driven by structural changes and that the relative stability of high-spin and low-spin states is sensitive to variations in the lattice constant. [, ]
Q15: How does cyanide bridging contribute to the magnetic properties of this compound(III) compounds?
A15: Cyanide (CN) ligands can act as bridging groups between metal centers, facilitating magnetic interactions. In this compound(III)-based compounds, these interactions can lead to a variety of magnetic behaviors, including ferromagnetism, ferrimagnetism, and metamagnetism. [, , , , , , ]
Q16: Can you give an example of a high-spin pentanuclear complex formed with this compound(III)?
A16: The reaction of this compound(III) with nickel(II) ions and specific bidentate organic ligands leads to the formation of pentanuclear complexes like [Cr(CN)6]2[Ni(IM2-py)2]3·7H2O. This complex features six organic radicals that couple ferromagnetically with the nickel(II) ions, resulting in a high-spin ground state (S = 9). []
Q17: What is photoinduced valence tautomerism, and how does it manifest in this compound(III)-based materials?
A17: Photoinduced valence tautomerism involves light-induced electron transfer between metal centers in a material, resulting in changes in their oxidation states and, consequently, their magnetic properties. Cobalt-iron Prussian blue analogs, including those with this compound(III) components, often exhibit this phenomenon. [, ]
Q18: Can you provide an example of a core@shell material where light induces changes in the magnetic properties of the shell?
A18: In core@shell particles of Rb0.24Co[Fe(CN)6]0.74@K0.10Co[Cr(CN)6]0.70·nH2O, light exposure triggers a charge transfer-induced spin transition (CTIST) in the rubidium cobalt hexacyanoferrate (RbCoFe) core. This transition, in turn, leads to structural changes in the potassium cobalt this compound (KCoCr) shell, modifying its magnetic properties through a magnetomechanical coupling effect. []
Q19: What role does this compound(III) play in chemical vapor generation for analytical chemistry?
A19: this compound(III) enhances the efficiency of cadmium vapor generation in inductively coupled plasma mass spectrometry (ICP-MS). The interaction of this compound(III) with sodium borohydride is believed to generate reactive intermediates that facilitate the formation of volatile cadmium species, improving the sensitivity of cadmium detection. []
Q20: How does gamma-ray irradiation affect cobalt-60 exchange in hexamminecobalt(III) this compound(III)?
A20: Gamma-ray irradiation of cobalt-60-doped hexamminecobalt(III) this compound(III) increases the percentage of cobalt(III) exchanged within the material. This exchange is attributed to radiation-induced defects, such as metal and ligand vacancies, which facilitate the exchange process. []
Q21: Are there examples of this compound(III) complexes exhibiting thermal CN-bridging reactions?
A21: Yes, double complex salts like [Co(H2O)2N4][Co(CN)6]·nH2O, where N4 represents various ligands, and [Co(H2O)2trien][Cr(CN)6]·2H2O, undergo thermal reactions involving the formation of CN bridges upon heating. This process is often accompanied by deaquation or deammonation reactions. []
Q22: What is significant about the compensation temperature observed in vanadium this compound-based magnets?
A22: The vanadium this compound-based ferrimagnet, K0.19V0.64(VIVO)0.36[CrIII(CN)6]0.73·7.5H2O, exhibits a remarkably high compensation temperature (Tcomp) of 175 K. This high Tcomp arises from the compensation of positive sublattice magnetization from chromium(III) by the negative sublattice magnetizations of vanadium(II) and vanadium(IV) ions within the material. []
体外研究产品的免责声明和信息
请注意,BenchChem 上展示的所有文章和产品信息仅供信息参考。 BenchChem 上可购买的产品专为体外研究设计,这些研究在生物体外进行。体外研究,源自拉丁语 "in glass",涉及在受控实验室环境中使用细胞或组织进行的实验。重要的是要注意,这些产品没有被归类为药物或药品,他们没有得到 FDA 的批准,用于预防、治疗或治愈任何医疗状况、疾病或疾病。我们必须强调,将这些产品以任何形式引入人类或动物的身体都是法律严格禁止的。遵守这些指南对确保研究和实验的法律和道德标准的符合性至关重要。