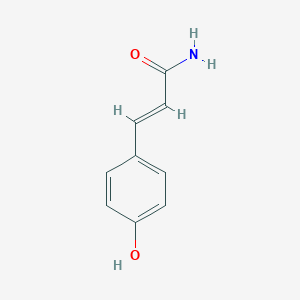
4-羟基肉桂酰胺
概述
描述
4-Hydroxycinnamamide, also known as 3-(4-hydroxyphenyl)acrylamide, is a compound with the molecular formula C9H9NO2 and a molecular weight of 163.2 g/mol . It is a derivative of cinnamic acid and is characterized by the presence of a hydroxyl group on the phenyl ring and an amide group at the end of the carbon chain. This compound is known for its antioxidant properties and its role as a specific inhibitor of tyrosine-specific protein kinases .
科学研究应用
4-Hydroxycinnamamide has a wide range of applications in scientific research:
Chemistry: Used as a precursor for synthesizing various derivatives with potential biological activities.
Biology: Studied for its role as an antioxidant and its ability to inhibit tyrosine-specific protein kinases.
Medicine: Investigated for its potential anticancer properties, particularly against leukemia cells.
作用机制
Target of Action
4-Hydroxycinnamamide is a potent inhibitor of tyrosine-specific protein kinases . These kinases are key components of signal transduction pathways and play crucial roles in cellular processes such as growth, differentiation, metabolism, and apoptosis . Additionally, 4-Hydroxycinnamamide has been identified as a Histone Deacetylase (HDAC) 1/3 dual inhibitor . HDACs are enzymes that remove acetyl groups from an ε-N-acetyl lysine amino acid on a histone, allowing the histones to wrap the DNA more tightly .
Mode of Action
4-Hydroxycinnamamide interacts with its targets by competing with the substrate protein for the tyrosine kinase binding site . This inhibitory activity results in a decrease in the phosphorylation of tyrosine residues, thereby disrupting the signal transduction pathways . As an HDAC inhibitor, it prevents the removal of acetyl groups from histones, leading to an “open” chromatin state that allows access of the transcription machinery to DNA .
Biochemical Pathways
The inhibition of tyrosine-specific protein kinases by 4-Hydroxycinnamamide affects multiple biochemical pathways involved in cell growth and differentiation . By inhibiting HDACs, it impacts the epigenetic regulation of gene expression . This can lead to changes in the expression of genes involved in cell cycle regulation, apoptosis, and other cellular functions .
Pharmacokinetics
Its ability to inhibit tyrosine-specific protein kinases and hdacs suggests that it can penetrate cell membranes and interact with intracellular targets .
Result of Action
The inhibition of tyrosine-specific protein kinases and HDACs by 4-Hydroxycinnamamide can lead to a variety of cellular effects. These include changes in cell growth and differentiation, induction of apoptosis, and alterations in gene expression . In particular, it has shown potent antitumor activity in vitro .
Action Environment
The action of 4-Hydroxycinnamamide can be influenced by various environmental factors. For instance, the pH and ion concentration of the cellular environment can impact the compound’s stability and efficacy . Additionally, factors such as temperature and light exposure can affect its stability during storage .
生化分析
Biochemical Properties
4-Hydroxycinnamamide has been found to inhibit tyrosine-specific protein kinases, which are enzymes that play a crucial role in many cellular processes . This compound interacts with these enzymes by competing with the substrate protein for the tyrosine kinase binding site .
Cellular Effects
The inhibition of tyrosine kinases by 4-Hydroxycinnamamide can have significant effects on cell function. Tyrosine kinases are involved in cell signaling pathways, gene expression, and cellular metabolism . By inhibiting these enzymes, 4-Hydroxycinnamamide can potentially influence these cellular processes.
Molecular Mechanism
4-Hydroxycinnamamide exerts its effects at the molecular level through its interactions with tyrosine kinases. It competes with the substrate protein for the tyrosine kinase binding site, thereby inhibiting the kinase’s activity . This can lead to changes in gene expression and other cellular processes.
Metabolic Pathways
4-Hydroxycinnamamide is involved in the metabolic pathway of tyrosine kinases. It interacts with these enzymes, potentially affecting metabolic flux or metabolite levels .
准备方法
Synthetic Routes and Reaction Conditions: 4-Hydroxycinnamamide can be synthesized through several methods. One common approach involves the amidation of 4-hydroxycinnamic acid. The process typically includes the following steps:
Acetylation: Protecting the phenolic hydroxyl group by converting it into an acetoxy group.
Chlorination: Activating the carboxyl group by converting it into an acyl chloride.
Amidation: Reacting the acyl chloride with an amine to form the amide bond.
Deacetylation: Removing the acetoxy protecting group to regenerate the hydroxyl group.
Industrial Production Methods: Industrial production of 4-Hydroxycinnamamide may involve similar steps but on a larger scale, with optimizations for yield and purity. The use of automated reactors and continuous flow systems can enhance the efficiency of the synthesis process.
化学反应分析
4-Hydroxycinnamamide undergoes various chemical reactions, including:
Oxidation: The hydroxyl group can be oxidized to form quinones.
Reduction: The amide group can be reduced to form amines.
Substitution: The hydroxyl group can participate in electrophilic aromatic substitution reactions.
Common Reagents and Conditions:
Oxidation: Reagents like potassium permanganate or chromium trioxide.
Reduction: Reagents like lithium aluminum hydride or sodium borohydride.
Substitution: Reagents like halogens or nitrating agents under acidic conditions.
Major Products:
Oxidation: Quinones.
Reduction: Amines.
Substitution: Halogenated or nitrated derivatives.
相似化合物的比较
- Cinnamamide
- N-methyl cinnamamide
- 3,4-Dihydroxycinnamamide
- Ferulamide
- N-caffeoyltyramine
生物活性
4-Hydroxycinnamamide (4-HCA) is a compound that has garnered attention due to its diverse biological activities, particularly in cancer research and as a potential therapeutic agent. This article synthesizes findings from various studies to provide a comprehensive overview of its biological activity, including mechanisms of action, efficacy in different cancer types, and potential therapeutic applications.
Chemical Structure and Properties
4-Hydroxycinnamamide, with the chemical formula CHNO, features a hydroxyl group attached to a cinnamamide structure. This unique configuration contributes to its biological properties, particularly its interaction with various biological targets.
Research has identified several mechanisms through which 4-HCA exerts its biological effects:
-
Inhibition of Tyrosine Kinases :
- A study highlighted that synthetic derivatives of 4-HCA, such as ST 638, inhibit tyrosine-specific protein kinases involved in oncogenic signaling pathways. These kinases include the epidermal growth factor receptor (EGFR) and various proto-oncogenes. The IC values for these inhibitors ranged from 1.1 µM for EGFR to 87 µM for pp60 v-src, indicating significant potency against these targets .
-
Histone Deacetylase Inhibition :
- Another study developed N-hydroxycinnamamide-based compounds that showed promising histone deacetylase (HDAC) inhibitory activity. Compounds derived from 4-HCA exhibited comparable or superior HDAC inhibition compared to established drugs like SAHA, suggesting potential applications in cancer therapy through epigenetic modulation .
- Anticancer Activity :
Efficacy in Cancer Models
The efficacy of 4-HCA has been evaluated in several preclinical models:
-
In Vitro Studies :
- In vitro assays have shown that 4-HCA derivatives can significantly reduce the viability of cancer cell lines, including those resistant to conventional therapies. For instance, one study reported that a specific derivative exhibited over 50% inhibition of tumor cell growth at concentrations as low as 1 µM .
- In Vivo Studies :
Case Studies and Research Findings
Several case studies have illustrated the biological activity of 4-HCA:
- Case Study: Antitumor Activity :
-
Case Study: HDAC Inhibition :
- Another investigation focused on the HDAC inhibitory activity of a series of N-hydroxycinnamamide derivatives. These compounds not only inhibited HDACs effectively but also displayed strong antiproliferative effects against cancer cell lines, suggesting their dual role as epigenetic modulators and cytotoxic agents .
Summary Table of Biological Activities
属性
IUPAC Name |
(E)-3-(4-hydroxyphenyl)prop-2-enamide | |
---|---|---|
Source | PubChem | |
URL | https://pubchem.ncbi.nlm.nih.gov | |
Description | Data deposited in or computed by PubChem | |
InChI |
InChI=1S/C9H9NO2/c10-9(12)6-3-7-1-4-8(11)5-2-7/h1-6,11H,(H2,10,12)/b6-3+ | |
Source | PubChem | |
URL | https://pubchem.ncbi.nlm.nih.gov | |
Description | Data deposited in or computed by PubChem | |
InChI Key |
DSMLJOHWFORNLY-ZZXKWVIFSA-N | |
Source | PubChem | |
URL | https://pubchem.ncbi.nlm.nih.gov | |
Description | Data deposited in or computed by PubChem | |
Canonical SMILES |
C1=CC(=CC=C1C=CC(=O)N)O | |
Source | PubChem | |
URL | https://pubchem.ncbi.nlm.nih.gov | |
Description | Data deposited in or computed by PubChem | |
Isomeric SMILES |
C1=CC(=CC=C1/C=C/C(=O)N)O | |
Source | PubChem | |
URL | https://pubchem.ncbi.nlm.nih.gov | |
Description | Data deposited in or computed by PubChem | |
Molecular Formula |
C9H9NO2 | |
Source | PubChem | |
URL | https://pubchem.ncbi.nlm.nih.gov | |
Description | Data deposited in or computed by PubChem | |
Molecular Weight |
163.17 g/mol | |
Source | PubChem | |
URL | https://pubchem.ncbi.nlm.nih.gov | |
Description | Data deposited in or computed by PubChem | |
CAS No. |
194940-15-3 | |
Record name | 2-Propenamide, 3-(4-hydroxyphenyl)-, (2E)- | |
Source | ChemIDplus | |
URL | https://pubchem.ncbi.nlm.nih.gov/substance/?source=chemidplus&sourceid=0194940153 | |
Description | ChemIDplus is a free, web search system that provides access to the structure and nomenclature authority files used for the identification of chemical substances cited in National Library of Medicine (NLM) databases, including the TOXNET system. | |
Record name | 2-PROPENAMIDE, 3-(4-HYDROXYPHENYL)-, (2E)- | |
Source | FDA Global Substance Registration System (GSRS) | |
URL | https://gsrs.ncats.nih.gov/ginas/app/beta/substances/AA6QNK95YZ | |
Description | The FDA Global Substance Registration System (GSRS) enables the efficient and accurate exchange of information on what substances are in regulated products. Instead of relying on names, which vary across regulatory domains, countries, and regions, the GSRS knowledge base makes it possible for substances to be defined by standardized, scientific descriptions. | |
Explanation | Unless otherwise noted, the contents of the FDA website (www.fda.gov), both text and graphics, are not copyrighted. They are in the public domain and may be republished, reprinted and otherwise used freely by anyone without the need to obtain permission from FDA. Credit to the U.S. Food and Drug Administration as the source is appreciated but not required. | |
Synthesis routes and methods
Procedure details
Retrosynthesis Analysis
AI-Powered Synthesis Planning: Our tool employs the Template_relevance Pistachio, Template_relevance Bkms_metabolic, Template_relevance Pistachio_ringbreaker, Template_relevance Reaxys, Template_relevance Reaxys_biocatalysis model, leveraging a vast database of chemical reactions to predict feasible synthetic routes.
One-Step Synthesis Focus: Specifically designed for one-step synthesis, it provides concise and direct routes for your target compounds, streamlining the synthesis process.
Accurate Predictions: Utilizing the extensive PISTACHIO, BKMS_METABOLIC, PISTACHIO_RINGBREAKER, REAXYS, REAXYS_BIOCATALYSIS database, our tool offers high-accuracy predictions, reflecting the latest in chemical research and data.
Strategy Settings
Precursor scoring | Relevance Heuristic |
---|---|
Min. plausibility | 0.01 |
Model | Template_relevance |
Template Set | Pistachio/Bkms_metabolic/Pistachio_ringbreaker/Reaxys/Reaxys_biocatalysis |
Top-N result to add to graph | 6 |
Feasible Synthetic Routes
Q1: What makes 4-hydroxycinnamamide a promising compound for scientific research?
A1: 4-Hydroxycinnamamide, isolated from the root bark of the plant Kleinhovia hospita Linn., exhibits notable toxicity against Artemia salina Leach (brine shrimp) with an IC50 of 180.53 µg/mL []. This finding suggests potential cytostatic activity against cancer cells, making it a promising candidate for further investigation.
Q2: What is the primary challenge in studying the biological activity of 4-hydroxycinnamamide?
A2: The concentration of 4-hydroxycinnamamide found naturally in the root bark of K. hospita Linn. is extremely low (around 1.6 ppm) []. Additionally, the compound is not commercially available. This scarcity necessitates efficient synthetic methods to produce sufficient quantities for in-depth biological and chemical studies.
Q3: How is 4-hydroxycinnamamide synthesized in the laboratory?
A3: A successful two-step synthesis of 4-hydroxycinnamamide starting from 4-hydroxycinnamic acid has been reported []. First, 4-hydroxycinnamic acid undergoes esterification with excess ethanol using sulfuric acid as a catalyst, yielding ethyl 4-hydroxycinnamate. Subsequently, ammonolysis of ethyl 4-hydroxycinnamate using concentrated ammonia produces 4-hydroxycinnamamide.
Q4: What spectroscopic techniques are employed to confirm the identity of synthesized 4-hydroxycinnamamide?
A4: The synthesized 4-hydroxycinnamamide and its intermediate, ethyl 4-hydroxycinnamate, are characterized using Fourier-transform infrared spectroscopy (FTIR) and nuclear magnetic resonance (NMR) spectroscopy []. These techniques provide detailed information about the functional groups and structure of the synthesized compounds, confirming their identity.
Q5: What is the significance of the double bond and the hydroxyl group in the structure of 4-hydroxycinnamamide derivatives for their inhibitory activity?
A5: Studies on the structure-activity relationship of 4-hydroxycinnamamide derivatives have revealed that the presence of the hydroxyl group at the 4-position and the double bond within the α-cyano-4-hydroxycinnamamide structure are crucial for potent inhibitory activity against tyrosine kinases [, ]. Modifications altering these structural features could lead to a decrease or loss of activity.
Q6: How do modifications at the 3 and 5 positions of the benzene ring in 4-hydroxycinnamamide derivatives impact their inhibitory activity?
A6: Introducing hydrophobic groups at the 3 and 5 positions of the benzene ring in α-cyano-4-hydroxycinnamamide derivatives enhances their inhibitory activity against tyrosine kinases []. This observation suggests that hydrophobic interactions at these positions play a role in the binding affinity and specificity of these compounds toward their target.
Q7: Could you provide an example of a potent 4-hydroxycinnamamide derivative and its mechanism of action?
A7: ST 638 (α-cyano-3-ethoxy-4-hydroxy-5-phenylthiomethylcinnamamide) is a potent 4-hydroxycinnamamide derivative that demonstrates significant inhibitory activity against various tyrosine kinases, including the epidermal growth factor (EGF) receptor kinase []. Kinetic studies suggest that ST 638 competitively inhibits the phosphorylation of exogenous substrates by binding to the tyrosine kinase active site. This binding prevents the natural substrate from interacting with the kinase, thus inhibiting downstream signaling pathways.
Q8: Beyond cancer, are there other potential applications for 4-hydroxycinnamamide derivatives?
A8: 4-Hydroxycinnamamide derivatives have shown promising results in inducing systemic acquired resistance (SAR) in rice against the parasitic nematode Meloidogyne graminicola []. This finding suggests a potential role for these compounds in developing environmentally friendly pest control strategies in agriculture.
Q9: How do 4-hydroxycinnamamide derivatives compare to salicylic acid in terms of inducing SAR in rice?
A9: N-hexyl-salicylamide, N-hexyl-2-hydroxycinnamamide, and N-hexyl-4-hydroxycinnamamide, all 4-hydroxycinnamamide derivatives, were found to be more effective than commercially available salicylic acid in inducing SAR in rice against Meloidogyne graminicola []. Notably, N-hexyl-salicylamide demonstrated the highest efficacy, suggesting a potential advantage of these derivatives over salicylic acid in this specific application.
体外研究产品的免责声明和信息
请注意,BenchChem 上展示的所有文章和产品信息仅供信息参考。 BenchChem 上可购买的产品专为体外研究设计,这些研究在生物体外进行。体外研究,源自拉丁语 "in glass",涉及在受控实验室环境中使用细胞或组织进行的实验。重要的是要注意,这些产品没有被归类为药物或药品,他们没有得到 FDA 的批准,用于预防、治疗或治愈任何医疗状况、疾病或疾病。我们必须强调,将这些产品以任何形式引入人类或动物的身体都是法律严格禁止的。遵守这些指南对确保研究和实验的法律和道德标准的符合性至关重要。