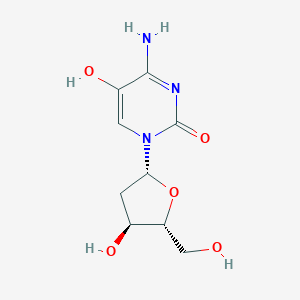
5-羟基-2'-脱氧胞苷
描述
5-Hydroxy-2'-deoxycytidine, also known as 5-Hydroxy-2'-deoxycytidine, is a useful research compound. Its molecular formula is C9H13N3O5 and its molecular weight is 243.22 g/mol. The purity is usually 95%.
The exact mass of the compound 2'-Deoxy-5-hydroxycytidine is unknown and the complexity rating of the compound is unknown. Its Medical Subject Headings (MeSH) category is Chemicals and Drugs Category - Carbohydrates - Glycosides - Nucleosides - Deoxyribonucleosides - Supplementary Records. The storage condition is unknown. Please store according to label instructions upon receipt of goods.
BenchChem offers high-quality 5-Hydroxy-2'-deoxycytidine suitable for many research applications. Different packaging options are available to accommodate customers' requirements. Please inquire for more information about 5-Hydroxy-2'-deoxycytidine including the price, delivery time, and more detailed information at info@benchchem.com.
科学研究应用
DNA 损伤与修复研究
5-羟基-2'-脱氧胞苷 (5-OHdC) 是氧化性 DNA 损伤的重要标志物和产物 . 它作为大肠杆菌内切核酸酶 III 和甲酰胺嘧啶 DNA N-糖基化酶等 DNA 修复酶的底物 . 涉及 5-OHdC 的研究可以提供对 DNA 修复机制和氧化应激在诱变中的作用的见解。
酶促底物特异性
该化合物用于研究各种 DNA 修复酶的底物特异性。 例如,5-OHdC 是已知的尿嘧啶 DNA N-糖基化酶的底物,参与碱基切除修复途径 . 这种应用对于理解酶的功能和设计用于治疗目的的抑制剂至关重要。
诱变研究
5-OHdC 被 DNA 聚合酶整合到 DNA 中,代替脱氧胞苷,这会导致突变 . 它用于诱变研究以了解核苷酸的氧化损伤如何导致遗传突变,从而有助于我们了解癌症等疾病过程 .
生化分析
在生化分析中,5-OHdC 用于监测 DNA 聚合酶的保真度和 DNA 修复酶的效率 . 这些分析是药物发现和开发的基础,特别是在识别可以调节这些酶的化合物方面。
癌症研究
在癌细胞中测量 5-OHdC 水平以了解氧化性 DNA 损伤在致癌作用中的作用 . 它也用于开发化学传感器,用于选择性检测和量化癌细胞中的修饰核苷 .
抗病毒研究
2'-脱氧-5-羟基胞苷已被研究用于治疗人类免疫缺陷病毒 (HIV)。 它通过靶向逆转录酶来抑制 HIV,并且对受损的 DNA 显示出反应性,这可以利用在抗病毒治疗中 .
分子生物学研究
该化合物用于研究 DNA 合成过程中依赖序列的错配。 它有助于理解由于氧化损伤而可能发生的转换突变和颠换突变的分子基础 <svg class="icon" height="16" p-id="1735" t="17092647886
作用机制
Target of Action
5-Hydroxy-2’-deoxycytidine (5-OHdC) and its analogue 5-hydroxy-2’-deoxyuridine (5-OHdU) are substrates for Escherichia coli endonuclease III and formamidopyrimidine DNA N-glycosylase (FPG). Additionally, 5-OHdU is a substrate for uracil DNA N-glycosylase . These enzymes play a crucial role in DNA repair and maintenance.
Mode of Action
The mode of action of 5-OHdC involves its interaction with the aforementioned enzymes. Endonuclease III removes 5-OHdC and 5-OHdU via a N-glycosylase/β-elimination reaction, FPG follows a N-glycosylase/β,δ-elimination reaction, and uracil N-glycosylase removes 5-OHdU by N-glycosylase action leaving behind an abasic site .
Biochemical Pathways
5-OHdC and 5-OHdU are major products of oxidative DNA damage with mutagenic potential . The biochemical pathways affected by 5-OHdC involve DNA repair mechanisms. The enzymes Escherichia coli endonuclease III, formamidopyrimidine DNA N-glycosylase, and uracil DNA N-glycosylase play a significant role in these pathways .
Result of Action
The primary result of 5-OHdC action is the repair of oxidative DNA damage. By serving as a substrate for DNA repair enzymes, 5-OHdC contributes to the maintenance of genomic integrity .
Action Environment
The action of 5-OHdC is influenced by the cellular environment, particularly the presence and activity of specific enzymes. Factors such as oxidative stress, which can increase the production of 5-OHdC, may also influence its action .
生化分析
Biochemical Properties
5-Hydroxy-2’-deoxycytidine interacts with several enzymes, proteins, and other biomolecules. It is a substrate for Escherichia coli endonuclease III and formamidopyrimidine DNA N-glycosylase . These enzymes remove 5-Hydroxy-2’-deoxycytidine via a N-glycosylase/β-elimination reaction . The nature of these interactions involves the oxidation of 5-Hydroxy-2’-deoxycytidine, which is a crucial step in the biochemical reactions involving this compound .
Cellular Effects
The effects of 5-Hydroxy-2’-deoxycytidine on various types of cells and cellular processes are significant. It influences cell function by interacting with DNA and RNA chains . It impacts cell signaling pathways, gene expression, and cellular metabolism. For instance, it has been used as a substrate for uracil DNA N-glycosylase and has been used to study the oxidation of DNA due to exposure to reactive oxygen species .
Molecular Mechanism
5-Hydroxy-2’-deoxycytidine exerts its effects at the molecular level through several mechanisms. It binds with biomolecules, inhibits or activates enzymes, and induces changes in gene expression. For example, it is incorporated into DNA during replication, where it inhibits DNA methyltransferases by creating permanent cross-links between the protein and the 5-aza-cytosine nucleobase .
Temporal Effects in Laboratory Settings
Over time in laboratory settings, the effects of 5-Hydroxy-2’-deoxycytidine change. It has been observed that the levels of 5-Hydroxy-2’-deoxycytidine in untreated commercial-grade calf thymus DNA increased substantially when exposed to various conditions, indicating that it is a major oxidative DNA damage product .
Metabolic Pathways
5-Hydroxy-2’-deoxycytidine is involved in several metabolic pathways. It interacts with enzymes like Escherichia coli endonuclease III and formamidopyrimidine DNA N-glycosylase
属性
IUPAC Name |
4-amino-5-hydroxy-1-[(2R,4S,5R)-4-hydroxy-5-(hydroxymethyl)oxolan-2-yl]pyrimidin-2-one | |
---|---|---|
Source | PubChem | |
URL | https://pubchem.ncbi.nlm.nih.gov | |
Description | Data deposited in or computed by PubChem | |
InChI |
InChI=1S/C9H13N3O5/c10-8-5(15)2-12(9(16)11-8)7-1-4(14)6(3-13)17-7/h2,4,6-7,13-15H,1,3H2,(H2,10,11,16)/t4-,6+,7+/m0/s1 | |
Source | PubChem | |
URL | https://pubchem.ncbi.nlm.nih.gov | |
Description | Data deposited in or computed by PubChem | |
InChI Key |
CKZJTNZSBMVFSU-UBKIQSJTSA-N | |
Source | PubChem | |
URL | https://pubchem.ncbi.nlm.nih.gov | |
Description | Data deposited in or computed by PubChem | |
Canonical SMILES |
C1C(C(OC1N2C=C(C(=NC2=O)N)O)CO)O | |
Source | PubChem | |
URL | https://pubchem.ncbi.nlm.nih.gov | |
Description | Data deposited in or computed by PubChem | |
Isomeric SMILES |
C1[C@@H]([C@H](O[C@H]1N2C=C(C(=NC2=O)N)O)CO)O | |
Source | PubChem | |
URL | https://pubchem.ncbi.nlm.nih.gov | |
Description | Data deposited in or computed by PubChem | |
Molecular Formula |
C9H13N3O5 | |
Source | PubChem | |
URL | https://pubchem.ncbi.nlm.nih.gov | |
Description | Data deposited in or computed by PubChem | |
DSSTOX Substance ID |
DTXSID00966652 | |
Record name | 1-(2-Deoxypentofuranosyl)-4-imino-1,4-dihydropyrimidine-2,5-diol | |
Source | EPA DSSTox | |
URL | https://comptox.epa.gov/dashboard/DTXSID00966652 | |
Description | DSSTox provides a high quality public chemistry resource for supporting improved predictive toxicology. | |
Molecular Weight |
243.22 g/mol | |
Source | PubChem | |
URL | https://pubchem.ncbi.nlm.nih.gov | |
Description | Data deposited in or computed by PubChem | |
CAS No. |
52278-77-0 | |
Record name | 5-Hydroxy-2′-deoxycytidine | |
Source | CAS Common Chemistry | |
URL | https://commonchemistry.cas.org/detail?cas_rn=52278-77-0 | |
Description | CAS Common Chemistry is an open community resource for accessing chemical information. Nearly 500,000 chemical substances from CAS REGISTRY cover areas of community interest, including common and frequently regulated chemicals, and those relevant to high school and undergraduate chemistry classes. This chemical information, curated by our expert scientists, is provided in alignment with our mission as a division of the American Chemical Society. | |
Explanation | The data from CAS Common Chemistry is provided under a CC-BY-NC 4.0 license, unless otherwise stated. | |
Record name | 5-Hydroxy-2'-deoxycytidine | |
Source | ChemIDplus | |
URL | https://pubchem.ncbi.nlm.nih.gov/substance/?source=chemidplus&sourceid=0052278770 | |
Description | ChemIDplus is a free, web search system that provides access to the structure and nomenclature authority files used for the identification of chemical substances cited in National Library of Medicine (NLM) databases, including the TOXNET system. | |
Record name | 1-(2-Deoxypentofuranosyl)-4-imino-1,4-dihydropyrimidine-2,5-diol | |
Source | EPA DSSTox | |
URL | https://comptox.epa.gov/dashboard/DTXSID00966652 | |
Description | DSSTox provides a high quality public chemistry resource for supporting improved predictive toxicology. | |
Record name | 2'-DEOXY-5-HYDROXYCYTIDINE | |
Source | FDA Global Substance Registration System (GSRS) | |
URL | https://gsrs.ncats.nih.gov/ginas/app/beta/substances/6JHE7FC4BX | |
Description | The FDA Global Substance Registration System (GSRS) enables the efficient and accurate exchange of information on what substances are in regulated products. Instead of relying on names, which vary across regulatory domains, countries, and regions, the GSRS knowledge base makes it possible for substances to be defined by standardized, scientific descriptions. | |
Explanation | Unless otherwise noted, the contents of the FDA website (www.fda.gov), both text and graphics, are not copyrighted. They are in the public domain and may be republished, reprinted and otherwise used freely by anyone without the need to obtain permission from FDA. Credit to the U.S. Food and Drug Administration as the source is appreciated but not required. | |
Retrosynthesis Analysis
AI-Powered Synthesis Planning: Our tool employs the Template_relevance Pistachio, Template_relevance Bkms_metabolic, Template_relevance Pistachio_ringbreaker, Template_relevance Reaxys, Template_relevance Reaxys_biocatalysis model, leveraging a vast database of chemical reactions to predict feasible synthetic routes.
One-Step Synthesis Focus: Specifically designed for one-step synthesis, it provides concise and direct routes for your target compounds, streamlining the synthesis process.
Accurate Predictions: Utilizing the extensive PISTACHIO, BKMS_METABOLIC, PISTACHIO_RINGBREAKER, REAXYS, REAXYS_BIOCATALYSIS database, our tool offers high-accuracy predictions, reflecting the latest in chemical research and data.
Strategy Settings
Precursor scoring | Relevance Heuristic |
---|---|
Min. plausibility | 0.01 |
Model | Template_relevance |
Template Set | Pistachio/Bkms_metabolic/Pistachio_ringbreaker/Reaxys/Reaxys_biocatalysis |
Top-N result to add to graph | 6 |
Feasible Synthetic Routes
Q1: How does 2'-deoxy-5-hydroxycytidine affect DNA replication and potentially contribute to mutations?
A1: Research suggests that 5-OHdC can be incorporated into DNA during replication by DNA polymerase, potentially acting as a premutagenic lesion. [, ] While 5-OHdCTP can be incorporated in place of dCTP and to a lesser extent dTTP, its presence in the DNA template leads to mispairing during replication. [] This mispairing varies depending on the surrounding DNA sequence context. For example, in one sequence context, dG was primarily incorporated opposite 5-OHdC, while in another, dC was predominantly incorporated. [] This sequence-dependent misincorporation suggests that 5-OHdC could lead to both C-->T transitions and C-->G transversions, potentially contributing to mutations. []
Q2: Can 2'-deoxy-5-hydroxycytidine be incorporated into DNA in the same way as canonical nucleotides?
A2: Research indicates that 5-OHdC can be incorporated into DNA, but not with the same fidelity as canonical nucleotides. Studies utilizing the Klenow fragment of Escherichia coli DNA polymerase I (lacking exonuclease activity) demonstrated that 5-OHdCTP can substitute for dCTP during DNA synthesis and, to a smaller extent, for dTTP. [] This suggests that DNA polymerase can recognize and utilize 5-OHdCTP as a substrate, albeit with a degree of infidelity.
体外研究产品的免责声明和信息
请注意,BenchChem 上展示的所有文章和产品信息仅供信息参考。 BenchChem 上可购买的产品专为体外研究设计,这些研究在生物体外进行。体外研究,源自拉丁语 "in glass",涉及在受控实验室环境中使用细胞或组织进行的实验。重要的是要注意,这些产品没有被归类为药物或药品,他们没有得到 FDA 的批准,用于预防、治疗或治愈任何医疗状况、疾病或疾病。我们必须强调,将这些产品以任何形式引入人类或动物的身体都是法律严格禁止的。遵守这些指南对确保研究和实验的法律和道德标准的符合性至关重要。