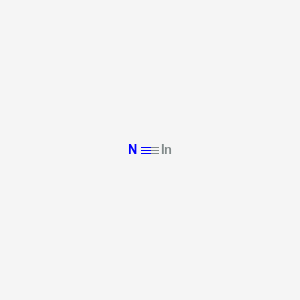
氮化铟
描述
Indium nitride (InN) is a small bandgap semiconductor material with potential applications in solar cells and high-speed electronics . Its bandgap has been established to be approximately 0.7 eV , although this value can vary with temperature . Compared to other group III-nitrides, InN stands out due to its narrow band gap, smallest effective mass, high electron mobility, and nearly invariant band gap across different temperatures. Researchers have been actively exploring its applications in field-effect transistors, lasers, solar cells, photodetectors, and thermoelectrics .
Synthesis Analysis
Indium nitride can be synthesized using various techniques, including metalorganic vapor phase epitaxy (MOVPE) and molecular beam epitaxy (MBE) . These growth methods allow precise control over the crystal structure and properties of InN .
Molecular Structure Analysis
- Additionally, two more possible phases—caesium chloride and nickel arsenide —have been explored. The crystal parameters for all six phases are compared with experimental and theoretical values. Band structures and density of states provide insights into their behavior in metal–insulator–semiconductor domains .
Physical And Chemical Properties Analysis
科学研究应用
High-Frequency Electronics
Indium nitride is a promising material for high-frequency electronics . Due to its high electron mobility, InN can facilitate the transmission of electrons at very high speeds, creating signals with extremely high frequencies. This makes it suitable for use in advanced communication technologies, potentially providing new frequencies for wireless data transfer .
depending on temperature, positions it as a potential material for . Its ability to utilize wavelengths up to 1900 nm can be advantageous in solar energy harvesting. .Light-Emitting Diodes (LEDs)
Indium-Gallium-Nitride (InGaN): -based LEDs are an area of active research. Optimizing the synthesis of InGaN can enhance the external quantum efficiency of blue LEDs, laser diodes, and quantum-dot solar cells. Novel approaches like adopting a microrod crystal structure and utilizing the piezo-phototronic effect have shown promising results in improving LED efficiency .
.Semiconductor-Superconductor Hybrids
InN can be used to create semiconductor-superconductor hybrids , such as Josephson-junction field-effect transistors. This application is particularly promising for magnetoelectronic devices where coupling superconductors to semiconductors is often problematic .
属性
IUPAC Name |
azanylidyneindigane | |
---|---|---|
Source | PubChem | |
URL | https://pubchem.ncbi.nlm.nih.gov | |
Description | Data deposited in or computed by PubChem | |
InChI |
InChI=1S/In.N | |
Source | PubChem | |
URL | https://pubchem.ncbi.nlm.nih.gov | |
Description | Data deposited in or computed by PubChem | |
InChI Key |
NWAIGJYBQQYSPW-UHFFFAOYSA-N | |
Source | PubChem | |
URL | https://pubchem.ncbi.nlm.nih.gov | |
Description | Data deposited in or computed by PubChem | |
Canonical SMILES |
N#[In] | |
Source | PubChem | |
URL | https://pubchem.ncbi.nlm.nih.gov | |
Description | Data deposited in or computed by PubChem | |
Molecular Formula |
InN | |
Record name | Indium nitride | |
Source | Wikipedia | |
URL | https://en.wikipedia.org/wiki/Indium_nitride | |
Description | Chemical information link to Wikipedia. | |
Source | PubChem | |
URL | https://pubchem.ncbi.nlm.nih.gov | |
Description | Data deposited in or computed by PubChem | |
DSSTOX Substance ID |
DTXSID7067112 | |
Record name | Indium nitride (InN) | |
Source | EPA DSSTox | |
URL | https://comptox.epa.gov/dashboard/DTXSID7067112 | |
Description | DSSTox provides a high quality public chemistry resource for supporting improved predictive toxicology. | |
Molecular Weight |
128.825 g/mol | |
Source | PubChem | |
URL | https://pubchem.ncbi.nlm.nih.gov | |
Description | Data deposited in or computed by PubChem | |
Physical Description |
Solid; [Merck Index] Grey powder; [MSDSonline] | |
Record name | Indium nitride | |
Source | Haz-Map, Information on Hazardous Chemicals and Occupational Diseases | |
URL | https://haz-map.com/Agents/9311 | |
Description | Haz-Map® is an occupational health database designed for health and safety professionals and for consumers seeking information about the adverse effects of workplace exposures to chemical and biological agents. | |
Explanation | Copyright (c) 2022 Haz-Map(R). All rights reserved. Unless otherwise indicated, all materials from Haz-Map are copyrighted by Haz-Map(R). No part of these materials, either text or image may be used for any purpose other than for personal use. Therefore, reproduction, modification, storage in a retrieval system or retransmission, in any form or by any means, electronic, mechanical or otherwise, for reasons other than personal use, is strictly prohibited without prior written permission. | |
Product Name |
Indium nitride | |
CAS RN |
25617-98-5 | |
Record name | Indium nitride | |
Source | CAS Common Chemistry | |
URL | https://commonchemistry.cas.org/detail?cas_rn=25617-98-5 | |
Description | CAS Common Chemistry is an open community resource for accessing chemical information. Nearly 500,000 chemical substances from CAS REGISTRY cover areas of community interest, including common and frequently regulated chemicals, and those relevant to high school and undergraduate chemistry classes. This chemical information, curated by our expert scientists, is provided in alignment with our mission as a division of the American Chemical Society. | |
Explanation | The data from CAS Common Chemistry is provided under a CC-BY-NC 4.0 license, unless otherwise stated. | |
Record name | Indium nitride | |
Source | ChemIDplus | |
URL | https://pubchem.ncbi.nlm.nih.gov/substance/?source=chemidplus&sourceid=0025617985 | |
Description | ChemIDplus is a free, web search system that provides access to the structure and nomenclature authority files used for the identification of chemical substances cited in National Library of Medicine (NLM) databases, including the TOXNET system. | |
Record name | Indium nitride (InN) | |
Source | EPA Chemicals under the TSCA | |
URL | https://www.epa.gov/chemicals-under-tsca | |
Description | EPA Chemicals under the Toxic Substances Control Act (TSCA) collection contains information on chemicals and their regulations under TSCA, including non-confidential content from the TSCA Chemical Substance Inventory and Chemical Data Reporting. | |
Record name | Indium nitride (InN) | |
Source | EPA DSSTox | |
URL | https://comptox.epa.gov/dashboard/DTXSID7067112 | |
Description | DSSTox provides a high quality public chemistry resource for supporting improved predictive toxicology. | |
Record name | Indium nitride | |
Source | European Chemicals Agency (ECHA) | |
URL | https://echa.europa.eu/substance-information/-/substanceinfo/100.042.831 | |
Description | The European Chemicals Agency (ECHA) is an agency of the European Union which is the driving force among regulatory authorities in implementing the EU's groundbreaking chemicals legislation for the benefit of human health and the environment as well as for innovation and competitiveness. | |
Explanation | Use of the information, documents and data from the ECHA website is subject to the terms and conditions of this Legal Notice, and subject to other binding limitations provided for under applicable law, the information, documents and data made available on the ECHA website may be reproduced, distributed and/or used, totally or in part, for non-commercial purposes provided that ECHA is acknowledged as the source: "Source: European Chemicals Agency, http://echa.europa.eu/". Such acknowledgement must be included in each copy of the material. ECHA permits and encourages organisations and individuals to create links to the ECHA website under the following cumulative conditions: Links can only be made to webpages that provide a link to the Legal Notice page. | |
Q & A
Q1: What are the challenges associated with synthesizing high-quality InN?
A1: InN synthesis poses several challenges due to its low dissociation temperature (~600 °C) [] and the large difference in partial pressures between typical precursors like trimethylindium (TMI) and ammonia (NH3) []. This often leads to difficulties in achieving stoichiometric growth and controlling nitrogen vacancies.
Q2: How does High-Pressure Chemical Vapor Deposition (HPCVD) address the challenges in InN growth?
A2: HPCVD offers a promising route for InN growth by injecting ambient nitrogen at super-atmospheric pressures, effectively suppressing InN surface dissociation at elevated temperatures []. This technique allows for a wider range of processing parameters, including higher growth temperatures, enabling improved crystalline quality.
Q3: Can alternative precursors be used for InN growth via Metal-Organic Vapor Phase Epitaxy (MOVPE)?
A3: While ammonia remains the preferred nitrogen precursor for MOVPE, research indicates that combining it with triethylindium can enhance growth rates while maintaining optimal crystalline quality [].
Q4: What is the role of plasma-assisted techniques in InN deposition?
A4: Plasma-assisted techniques, like plasma-assisted molecular beam epitaxy (PAMBE), are instrumental in activating nitrogen, a crucial factor for efficient InN growth [, ]. They provide a source of reactive nitrogen species, enabling the growth of high-quality InN films even at lower temperatures.
Q5: How does the choice of substrate influence InN growth?
A5: Lattice mismatch between InN and commonly used substrates like sapphire necessitates strategies like double-step growth processes []. The use of GaN/sapphire templates offers improved lattice matching, leading to better crystal quality compared to direct growth on sapphire substrates [].
Q6: What are the lattice parameters of wurtzite InN?
A7: Rietveld refinement studies have determined the lattice parameters of wurtzite InN to be a = 3.5366 ± 0.0005 Å and c = 5.7009 ± 0.0005 Å, with a c/a ratio of 1.612 ± 0.0005 []. These parameters can vary slightly depending on growth conditions and the presence of strain.
Q7: How does temperature affect the lattice parameters of InN?
A8: X-ray diffraction studies within the temperature range of 105-295 K reveal a smooth, almost linear increase in both a and c lattice parameters with rising temperature []. This behavior highlights the importance of considering temperature effects in InN-based device design.
Q8: What is the significance of the free structural parameter 'u' in wurtzite InN?
A9: The free structural parameter 'u', representing the anion displacement along the c-axis, provides insights into the bonding characteristics and structural stability of wurtzite crystals. For InN, 'u' has been experimentally determined to be 0.3769(14) [].
Q9: What is the band gap energy of InN, and why is it of interest?
A10: The band gap energy of InN has been a subject of debate. While initially believed to be around 1.9 eV, recent studies point to a much narrower band gap, potentially as low as 0.7 eV [, ]. This narrow band gap makes InN attractive for infrared optoelectronics, including high-efficiency solar cells and infrared detectors.
Q10: What factors influence the observed band gap energy of InN?
A11: Factors like film quality, strain, and the presence of impurities, particularly oxygen, can significantly influence the measured band gap energy of InN [, ]. Accurate determination of the intrinsic band gap requires careful material characterization and analysis.
Q11: How does quantum confinement affect the optical properties of InN nanostructures?
A12: Quantum confinement effects become significant in nanoscale InN structures, such as nanowires. This can lead to a blueshift in the emitted light, pushing the emission into the visible range for nanowires with diameters around 1 nm []. This property opens avenues for developing InN-based LEDs emitting green or cyan light [].
Q12: What is the dominant type of conductivity in InN, and what contributes to it?
A13: Indium Nitride exhibits a strong propensity for n-type conductivity, primarily attributed to the presence of native point defects, particularly indium vacancies (In vacancies) and their complexes [, ]. These defects act as donors, contributing to the unintentional n-type behavior.
Q13: How do growth conditions influence the formation of In vacancies in InN?
A14: Studies on In-polar InN grown by plasma-assisted MBE suggest that stoichiometric conditions during growth might not be the primary factor influencing In vacancy formation []. Instead, optimizing the buffer layer appears to play a more significant role, highlighting the importance of structural factors in defect control [].
Q14: What is the role of positron annihilation spectroscopy in studying defects in InN?
A15: Positron annihilation spectroscopy serves as a powerful tool for characterizing vacancy-type defects in InN [, , ]. By analyzing the annihilation characteristics of positrons trapped at defects, researchers can gain valuable insights into the types and concentrations of these defects.
Q15: How do thermal annealing and irradiation affect defects in InN?
A16: Research indicates that rapid thermal annealing after He-irradiation can induce significant changes in the defect structure of InN []. This process can lead to the restructuring of In vacancies near the film-substrate interface, potentially influencing the material's electrical properties [].
Q16: What types of InN nanostructures have been synthesized, and what are their potential applications?
A17: Various InN nanostructures, including nanowires [, , , , ], nanoparticles [], and microtubes [], have been successfully synthesized. These nanostructures exhibit unique properties that make them suitable for applications like high-efficiency LEDs [, ], near-infrared photodetectors [], and electrochromic devices [].
Q17: How does the morphology of InN nanostructures influence their properties?
A18: The morphology of InN nanostructures plays a crucial role in determining their properties. For instance, in InN nanowires, the shape of the cross-section can affect the emitted light color due to quantum confinement effects []. Controlling the morphology is, therefore, essential for tailoring InN nanostructures for specific applications.
Q18: What are the advantages of using InN nanocrystals in near-infrared photodetectors?
A19: InN nanocrystals offer high photoresponsivity in the near-infrared (NIR) range, making them attractive for NIR detection []. Their size-tunable optical properties and compatibility with large-area fabrication techniques further enhance their potential for various sensing applications.
Q19: How can the surface of InN be modified for improved device performance?
A20: Surface treatments, such as chemical etching [], can be employed to control the surface morphology and properties of InN. For example, etching InN with alkaline solutions like KOH can produce smooth, etch-pit-free surfaces, enhancing its suitability for device fabrication [].
Q20: What are the potential advantages of InN over other III-nitride materials?
A21: Compared to other III-nitrides like GaN, InN boasts a higher theoretical electron mobility and a narrower band gap [, ]. These properties make InN particularly attractive for high-speed electronic devices and applications requiring infrared light emission or detection.
体外研究产品的免责声明和信息
请注意,BenchChem 上展示的所有文章和产品信息仅供信息参考。 BenchChem 上可购买的产品专为体外研究设计,这些研究在生物体外进行。体外研究,源自拉丁语 "in glass",涉及在受控实验室环境中使用细胞或组织进行的实验。重要的是要注意,这些产品没有被归类为药物或药品,他们没有得到 FDA 的批准,用于预防、治疗或治愈任何医疗状况、疾病或疾病。我们必须强调,将这些产品以任何形式引入人类或动物的身体都是法律严格禁止的。遵守这些指南对确保研究和实验的法律和道德标准的符合性至关重要。