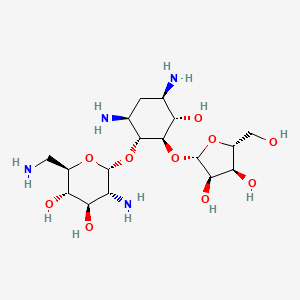
Ribostamycin
描述
Ribostamycin is an aminoglycoside-aminocyclitol antibiotic isolated from the bacterium Streptomyces ribosidificus. It is composed of three ring subunits: 2-deoxystreptamine, neosamine C, and ribose . This compound is recognized for its broad-spectrum antibiotic activity and is listed as a critically important antimicrobial by the World Health Organization .
准备方法
Synthetic Routes and Reaction Conditions: The biosynthesis of ribostamycin begins with D-glucose, which is phosphorylated to form glucose-6-phosphate. This undergoes a series of enzymatic reactions involving transamination, oxidation, and glycosylation to form the final product . The enzymes involved include rbmA, rbmB, rbmC, rbmD, racJ, rbmG, and rbmH .
Industrial Production Methods: Industrial production of this compound typically involves fermentation using Streptomyces ribosidificus. The fermentation broth is then processed to extract and purify this compound . High-purity this compound can be obtained by adjusting the pH of the fermentation liquor and controlling the temperature and other conditions during the extraction process .
化学反应分析
Types of Reactions: Ribostamycin undergoes various chemical reactions, including glycosylation, transamination, and oxidation .
Common Reagents and Conditions:
Glycosylation: Utilizes uridine diphosphate N-acetylglucosamine (UDP-GlcNAc) as a sugar donor.
Transamination: Involves L-glutamine and pyridoxal phosphate (PLP) as reagents.
Oxidation: Catalyzed by specific enzymes such as rbmC.
Major Products Formed: The major products formed from these reactions include intermediates like 2-deoxy-scyllo-inosose, 2-deoxy-scyllo-inosamine, and neamine, which eventually lead to the formation of this compound .
科学研究应用
Minimum Inhibitory Concentration (MIC)
Ribostamycin exhibits varying degrees of effectiveness against different bacterial strains. The minimum inhibitory concentration (MIC) is a critical measure used to evaluate the potency of antibiotics. Recent studies have reported the following MIC values for this compound:
Bacterial Strain | MIC (μM) |
---|---|
Escherichia coli (various strains) | 0.9 – 29.0 |
Haemophilus influenzae | 0.5 |
Staphylococcus aureus | 57.9 – 115.8 |
Pseudomonas aeruginosa | >115.8 |
Enterococcus faecalis | >115.8 |
These values indicate that this compound is particularly effective against certain strains of E. coli and H. influenzae, while showing limited efficacy against resistant strains like Staphylococcus aureus and Pseudomonas aeruginosa .
Combination Therapy
Recent research has demonstrated that the efficacy of this compound can be significantly enhanced when used in combination with ethylenediaminetetraacetic acid (EDTA). This combination therapy resulted in an eight-fold improvement in MIC values against resistant bacterial strains, suggesting that EDTA may serve as a valuable adjuvant to increase the clinical utility of this compound .
Gastrointestinal Infections
This compound has shown promise in treating gastrointestinal infections caused by various pathogens, particularly those linked to foodborne illnesses. The Centers for Disease Control and Prevention reports that infections caused by E. coli are a significant public health concern, with this compound demonstrating effective antibacterial activity against several strains associated with these infections . Its low absorption from the gastrointestinal tract minimizes systemic side effects, making it an attractive option for localized treatment .
Resistance Management
The growing concern over antibiotic resistance necessitates the exploration of alternative therapies. This compound's unique mechanism of action and its effectiveness against certain resistant strains make it a candidate for further investigation in managing infections caused by multidrug-resistant bacteria .
Nephrotoxicity Evaluation
One significant advantage of this compound is its low nephrotoxicity compared to other aminoglycosides. In vitro studies have indicated that this compound exhibits minimal toxicity towards kidney cells, with an IC50 greater than 100 mM . This characteristic enhances its appeal as a therapeutic agent, particularly for patients who may be at risk for renal complications.
Research on Biosynthesis
Understanding the biosynthesis of this compound can aid in developing new derivatives with improved efficacy and reduced resistance profiles. Current research focuses on elucidating the pathways involved in its synthesis, which may lead to novel antibiotic formulations .
作用机制
Ribostamycin is part of the aminoglycoside class of antibiotics, which includes other compounds such as neomycin, butirosin, and xylostasin . Compared to these, this compound is unique due to its specific ring subunits and its biosynthetic pathway . While all these compounds share a common mechanism of action, this compound’s structure allows for specific interactions with bacterial ribosomal RNA, making it particularly effective against certain bacterial strains .
相似化合物的比较
- Neomycin
- Butirosin
- Xylostasin
Ribostamycin’s broad-spectrum activity and its critical role in combating bacterial infections highlight its importance in both clinical and research settings.
生物活性
Ribostamycin is an aminoglycoside antibiotic derived from the actinobacterium Streptomyces ribosidificus. This compound exhibits a unique structure and mechanism of action, contributing to its biological activity against various bacterial pathogens. This article explores the biological activity of this compound, focusing on its antimicrobial properties, efficacy against resistant strains, and potential clinical applications.
Antimicrobial Properties
This compound's antimicrobial activity has been extensively studied in vitro and in vivo. It demonstrates varying levels of effectiveness against Gram-positive and Gram-negative bacteria. Below is a summary of its Minimum Inhibitory Concentration (MIC) values against selected bacterial strains.
Bacterial Strain | MIC (μM) | Activity Level |
---|---|---|
Escherichia coli (various strains) | 0.9 – 29.0 | Moderate to High |
Haemophilus influenzae | 0.5 | High |
Staphylococcus epidermidis | 0.1 – 13.7 | High |
Staphylococcus aureus | >115.8 | Very Weak |
Pseudomonas aeruginosa | >115.8 | Very Weak |
This compound exhibits potent activity against certain strains of E. coli, particularly when combined with ethylenediaminetetraacetic acid (EDTA), which enhances its efficacy significantly, achieving an 8-fold improvement in MIC values for some strains .
Comparative Efficacy
In comparative studies, this compound has shown comparable or slightly weaker in vitro activity than established antibiotics like gentamicin and ampicillin against Gram-positive bacteria. However, it has demonstrated superior efficacy against certain gentamicin-resistant strains of Klebsiella pneumoniae that harbor aminoglycoside-modifying enzymes .
In vivo studies indicated that this compound had a lower effective dose (ED50) than expected based on its MIC values, suggesting a rapid bactericidal action at high concentrations . The following table summarizes the comparative effectiveness of this compound and other antibiotics:
Antibiotic | In Vitro Activity | In Vivo Activity |
---|---|---|
This compound | Comparable to ampicillin | Weaker than gentamicin |
Gentamicin | Strong | Strong |
Ampicillin | Moderate | Comparable to this compound |
Lincomycin | Weak | Comparable to this compound |
Case Studies and Research Findings
Several studies have highlighted the potential of this compound in treating infections caused by resistant bacterial strains:
- Pregnancy Outcomes : A study evaluated the outcomes of women inadvertently exposed to this compound during early pregnancy, indicating no significant adverse effects on fetal development compared to control subjects .
- Combination Therapy : Research demonstrated that combining this compound with EDTA not only enhanced its antibacterial activity but also suggested a strategic approach for overcoming antibiotic resistance in certain bacterial infections .
- Spectrum of Activity : this compound has been shown to be particularly effective against gastrointestinal pathogens, making it a candidate for treating infections linked to gastrointestinal disturbances .
常见问题
Basic Research Questions
Q. What are the key structural features of ribostamycin that influence its antimicrobial activity?
this compound's structure (C₁₇H₃₄N₄O₁₀·xH₂SO₄) includes a 2-deoxystreptamine core and a ribose moiety, critical for rRNA binding in bacterial ribosomes . The absence of a C3′ hydroxyl group enhances resistance to aminoglycoside-modifying enzymes (AMEs), a feature shared with tobramycin and bekanamycin . Structural studies using mass spectrometry (MS) reveal that pseudotrisaccharide configurations (e.g., ribose glycosylation at the 5′′-position) determine binding specificity to prokaryotic rRNA A-sites .
Q. What experimental methods are recommended for assessing this compound's minimum inhibitory concentration (MIC)?
MIC assays should use standardized broth microdilution methods under aerobic conditions, with bacterial inocula adjusted to 1–5 × 10⁵ CFU/mL. For example, this compound exhibits an MIC of 18.7 mg/L against Borrelia burgdorferi . Include controls for pH (6–8) and cation concentration (Mg²⁺/Ca²⁺), as these factors influence aminoglycoside uptake .
Q. How should this compound be handled to ensure stability in laboratory settings?
Store this compound sulfate as a lyophilized powder at -20°C in airtight, light-protected containers. For aqueous solutions, use sterile deionized water (pH 6.5–7.5) and avoid repeated freeze-thaw cycles. Solubility exceeds 50 mg/mL in water but is negligible in ethanol or ether .
Advanced Research Questions
Q. How can mass spectrometry (MS) elucidate this compound's binding specificity to rRNA targets?
High-resolution MS with neutral mass tags enables simultaneous analysis of this compound interactions with multiple RNA subdomains. MS-MS protection assays identify binding sites by fragmenting this compound-rRNA complexes and mapping protection patterns. For example, base substitutions at positions 1408 (A→G) or 1409–1491 (mismatched pairs) in prokaryotic rRNA enhance binding affinity . This method quantitatively distinguishes this compound from paromomycin, which preferentially binds eukaryotic rRNA .
Q. What genetic engineering approaches exist for modifying this compound's biosynthetic pathway?
this compound biosynthesis in Streptomyces ribosidificus involves two key enzymes: BtrL (a phosphoribosyltransferase) and BtrP (a phosphatase). Heterologous expression of these enzymes in S. venezuelae enables ribosylation of neamine to form 5′′-phosphothis compound, followed by dephosphorylation to this compound . Site-directed mutagenesis of aprD3 (a radical SAM oxidoreductase) and aprD4 (a dehydrogenase) can eliminate 3′-hydroxyl groups, producing derivatives like nebramine .
Q. How can researchers resolve contradictions between this compound's PDI chaperone inhibition and lack of direct enzymatic activity?
this compound inhibits the molecular chaperone activity of protein disulfide isomerase (PDI) at a 100:1 molar ratio but does not affect its enzymatic activity. To confirm this, use fluorescence quenching assays with PDI-bound 8-anilino-1-naphthalenesulfonate (ANS) and compare results with enzymatic activity assays (e.g., insulin reduction). Structural modeling suggests this compound binds to PDI's hydrophobic substrate-binding domain, disrupting client protein folding .
Q. What statistical considerations are critical when designing this compound toxicity studies?
For renal or ototoxicity assessments, use longitudinal designs with repeated-measures ANOVA to account for time-dependent effects. In guinea pig models, this compound's low cochlear fluid concentration (vs. gentamicin) correlates with reduced ototoxicity, requiring a sample size of ≥15 animals/group to achieve 80% power (α=0.05) . Report exact p-values and confidence intervals to avoid overinterpreting "significant" results .
Q. Methodological Guidance
属性
IUPAC Name |
5-amino-2-(aminomethyl)-6-[4,6-diamino-2-[3,4-dihydroxy-5-(hydroxymethyl)oxolan-2-yl]oxy-3-hydroxycyclohexyl]oxyoxane-3,4-diol | |
---|---|---|
Details | Computed by Lexichem TK 2.7.0 (PubChem release 2021.05.07) | |
Source | PubChem | |
URL | https://pubchem.ncbi.nlm.nih.gov | |
Description | Data deposited in or computed by PubChem | |
InChI |
InChI=1S/C17H34N4O10/c18-2-6-10(24)12(26)8(21)16(28-6)30-14-5(20)1-4(19)9(23)15(14)31-17-13(27)11(25)7(3-22)29-17/h4-17,22-27H,1-3,18-21H2 | |
Details | Computed by InChI 1.0.6 (PubChem release 2021.05.07) | |
Source | PubChem | |
URL | https://pubchem.ncbi.nlm.nih.gov | |
Description | Data deposited in or computed by PubChem | |
InChI Key |
NSKGQURZWSPSBC-UHFFFAOYSA-N | |
Details | Computed by InChI 1.0.6 (PubChem release 2021.05.07) | |
Source | PubChem | |
URL | https://pubchem.ncbi.nlm.nih.gov | |
Description | Data deposited in or computed by PubChem | |
Canonical SMILES |
C1C(C(C(C(C1N)OC2C(C(C(C(O2)CN)O)O)N)OC3C(C(C(O3)CO)O)O)O)N | |
Details | Computed by OEChem 2.3.0 (PubChem release 2021.05.07) | |
Source | PubChem | |
URL | https://pubchem.ncbi.nlm.nih.gov | |
Description | Data deposited in or computed by PubChem | |
Molecular Formula |
C17H34N4O10 | |
Details | Computed by PubChem 2.1 (PubChem release 2021.05.07) | |
Source | PubChem | |
URL | https://pubchem.ncbi.nlm.nih.gov | |
Description | Data deposited in or computed by PubChem | |
DSSTOX Substance ID |
DTXSID80860346 | |
Record name | 4,6-Diamino-3-hydroxy-2-(pentofuranosyloxy)cyclohexyl 2,6-diamino-2,6-dideoxyhexopyranoside | |
Source | EPA DSSTox | |
URL | https://comptox.epa.gov/dashboard/DTXSID80860346 | |
Description | DSSTox provides a high quality public chemistry resource for supporting improved predictive toxicology. | |
Molecular Weight |
454.5 g/mol | |
Details | Computed by PubChem 2.1 (PubChem release 2021.05.07) | |
Source | PubChem | |
URL | https://pubchem.ncbi.nlm.nih.gov | |
Description | Data deposited in or computed by PubChem | |
CAS No. |
50474-67-4, 25546-65-0 | |
Record name | Xylostasin | |
Source | DTP/NCI | |
URL | https://dtp.cancer.gov/dtpstandard/servlet/dwindex?searchtype=NSC&outputformat=html&searchlist=236658 | |
Description | The NCI Development Therapeutics Program (DTP) provides services and resources to the academic and private-sector research communities worldwide to facilitate the discovery and development of new cancer therapeutic agents. | |
Explanation | Unless otherwise indicated, all text within NCI products is free of copyright and may be reused without our permission. Credit the National Cancer Institute as the source. | |
Record name | ribostamycin | |
Source | DTP/NCI | |
URL | https://dtp.cancer.gov/dtpstandard/servlet/dwindex?searchtype=NSC&outputformat=html&searchlist=138925 | |
Description | The NCI Development Therapeutics Program (DTP) provides services and resources to the academic and private-sector research communities worldwide to facilitate the discovery and development of new cancer therapeutic agents. | |
Explanation | Unless otherwise indicated, all text within NCI products is free of copyright and may be reused without our permission. Credit the National Cancer Institute as the source. | |
Retrosynthesis Analysis
AI-Powered Synthesis Planning: Our tool employs the Template_relevance Pistachio, Template_relevance Bkms_metabolic, Template_relevance Pistachio_ringbreaker, Template_relevance Reaxys, Template_relevance Reaxys_biocatalysis model, leveraging a vast database of chemical reactions to predict feasible synthetic routes.
One-Step Synthesis Focus: Specifically designed for one-step synthesis, it provides concise and direct routes for your target compounds, streamlining the synthesis process.
Accurate Predictions: Utilizing the extensive PISTACHIO, BKMS_METABOLIC, PISTACHIO_RINGBREAKER, REAXYS, REAXYS_BIOCATALYSIS database, our tool offers high-accuracy predictions, reflecting the latest in chemical research and data.
Strategy Settings
Precursor scoring | Relevance Heuristic |
---|---|
Min. plausibility | 0.01 |
Model | Template_relevance |
Template Set | Pistachio/Bkms_metabolic/Pistachio_ringbreaker/Reaxys/Reaxys_biocatalysis |
Top-N result to add to graph | 6 |
Feasible Synthetic Routes
体外研究产品的免责声明和信息
请注意,BenchChem 上展示的所有文章和产品信息仅供信息参考。 BenchChem 上可购买的产品专为体外研究设计,这些研究在生物体外进行。体外研究,源自拉丁语 "in glass",涉及在受控实验室环境中使用细胞或组织进行的实验。重要的是要注意,这些产品没有被归类为药物或药品,他们没有得到 FDA 的批准,用于预防、治疗或治愈任何医疗状况、疾病或疾病。我们必须强调,将这些产品以任何形式引入人类或动物的身体都是法律严格禁止的。遵守这些指南对确保研究和实验的法律和道德标准的符合性至关重要。