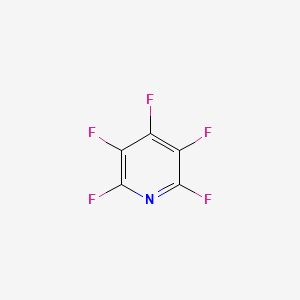
五氟吡啶
描述
Pentafluoropyridine, also known as perfluoropyridine, is a significant perfluoroaromatic compound with the chemical formula C5F5N. It features a six-membered aromatic ring where five fluorine atoms replace all hydrogen atoms on the carbon atoms.
Synthetic Routes and Reaction Conditions:
Method One: The synthesis of pentafluoropyridine was first reported in the early 1960s, involving the defluorination of perfluoropiperidine. Perfluoropiperidine was prepared by reacting pyridine with anhydrous hydrogen fluoride in an electrochemical synthesis at high temperatures with metals like iron or nickel. Subsequently, pentafluoropyridine was obtained by chromatographic separation.
Method Two: In 1964 and 1965, Chambers et al. and Banks et al. respectively published similar synthesis methods for pentafluoropyridine. The authors heated pentachloropyridine and anhydrous potassium fluoride in a high-pressure vessel to produce pentafluoropyridine. Pentachloropyridine was prepared by reacting pyridine with phosphorus pentachloride. This method produced a mixture of products, which could be separated by distillation. The total amount of halogenated products was about 90%, and the product ratio could be adjusted by changing the temperature and reaction time.
Industrial Production Methods:
- The method involving the reaction of pentachloropyridine with anhydrous potassium fluoride remains the gold standard for commercial synthesis of pentafluoropyridine due to its high yield and efficiency .
Types of Reactions:
Nucleophilic Substitution: Pentafluoropyridine undergoes nucleophilic aromatic substitution reactions (SNAr) with various nucleophiles such as oxygen, nitrogen, and sulfur-centered nucleophiles.
CF Bond Activation: This compound is involved in CF bond activation, leading to various chemical transformations.
Photo-Oxidation-Reduction Reactions: Pentafluoropyridine can participate in photo-oxidation-reduction reactions.
Common Reagents and Conditions:
- Reactions with amidoximes under basic conditions in dry acetonitrile result in the formation of substituted imidamide systems .
- The use of hydrogen halides in tetrahydrothiophene dioxide leads to the replacement of fluorine ortho and para to ring nitrogen by other halogens .
Major Products:
科学研究应用
Pentafluoropyridine has a wide range of applications in scientific research:
作用机制
Target of Action
Pentafluoropyridine is a highly reactive electrophile . It primarily targets nucleophilic peptide side chains, such as cysteine, tyrosine, serine, and lysine . These amino acids play crucial roles in protein structure and function, and their modification can significantly alter the properties of the protein.
Mode of Action
Pentafluoropyridine undergoes aromatic nucleophilic substitution reactions with its targets . The compound interacts with the oxygen site of amidoximes, resulting in the formation of substituted imidamide systems . This reaction primarily occurs at the 4-position of the pyridine ring .
Biochemical Pathways
For instance, it has been utilized in the synthesis of substituted 3,5-difluoropyridines, which have been investigated as new antithrombotic drugs .
Result of Action
The molecular and cellular effects of pentafluoropyridine’s action depend on the specific targets and the nature of the modifications. For example, the compound’s reaction with amidoximes leads to the formation of substituted imidamide systems . These systems could potentially alter protein function and cellular processes.
Action Environment
Environmental factors can influence the action, efficacy, and stability of pentafluoropyridine. For instance, the compound’s reactivity with nucleophiles can be affected by conditions such as temperature and solvent . Moreover, the presence of other reactive species in the environment could potentially compete with the compound’s targets, influencing its mode of action.
生化分析
Biochemical Properties
Pentafluoropyridine plays a significant role in biochemical reactions, particularly in nucleophilic substitution processes. It interacts with various enzymes, proteins, and other biomolecules. For instance, pentafluoropyridine reacts with amidoximes under basic conditions, leading to the formation of substituted imidamide systems . The interaction occurs at the 4-position of the pyridine ring by the oxygen site of amidoximes . Additionally, pentafluoropyridine can undergo arylation reactions with nucleophilic peptide side chains such as cysteine, tyrosine, serine, and lysine . These interactions highlight the compound’s versatility in biochemical applications.
Cellular Effects
Pentafluoropyridine influences various cellular processes and functions. It affects cell signaling pathways, gene expression, and cellular metabolism. The compound’s electrophilic nature allows it to interact with nucleophilic sites within cells, potentially altering cellular functions. For example, pentafluoropyridine’s interaction with peptide side chains can modify protein functions and signaling pathways . These modifications can lead to changes in gene expression and metabolic processes, impacting overall cellular health and function.
Molecular Mechanism
The molecular mechanism of pentafluoropyridine involves its ability to act as an electrophile, undergoing nucleophilic substitution reactions. It binds to nucleophilic sites on biomolecules, such as the oxygen site of amidoximes, leading to the formation of new chemical bonds . This binding can result in enzyme inhibition or activation, depending on the specific biomolecule involved. Additionally, pentafluoropyridine can influence gene expression by modifying transcription factors or other regulatory proteins, thereby altering the expression of specific genes.
Temporal Effects in Laboratory Settings
In laboratory settings, the effects of pentafluoropyridine can change over time. The compound’s stability and degradation are crucial factors in its long-term effects on cellular function. Studies have shown that pentafluoropyridine can form stable products under specific conditions, such as concentrated acetonitrile and reflux . Its reactivity may decrease over time in diluted conditions, leading to different reaction products . Long-term exposure to pentafluoropyridine in in vitro or in vivo studies may result in cumulative effects on cellular functions, necessitating careful monitoring of its stability and degradation.
Dosage Effects in Animal Models
The effects of pentafluoropyridine vary with different dosages in animal models. At lower doses, the compound may exhibit beneficial effects, such as enzyme activation or enhanced cellular functions. At higher doses, pentafluoropyridine can become toxic, leading to adverse effects on cellular health and function. Studies have shown that high doses of pentafluoropyridine can cause significant changes in metabolic processes and gene expression, potentially resulting in toxicity . Therefore, it is essential to determine the optimal dosage range for its safe and effective use in biochemical applications.
Metabolic Pathways
Pentafluoropyridine is involved in various metabolic pathways, interacting with enzymes and cofactors. Its nucleophilic substitution reactions can influence metabolic flux and metabolite levels. For example, pentafluoropyridine’s interaction with amidoximes can lead to the formation of novel pyridooxadiazine systems . These interactions can alter the metabolic pathways within cells, affecting the overall metabolic balance and function.
Subcellular Localization
Pentafluoropyridine’s subcellular localization is determined by its targeting signals and post-translational modifications. The compound can be directed to specific compartments or organelles within cells, influencing its activity and function. For instance, pentafluoropyridine’s interaction with nucleophilic peptide side chains can lead to its localization in specific cellular regions . These localization patterns can affect the compound’s biochemical properties and overall impact on cellular functions.
相似化合物的比较
Tetrafluoropyrimidine: Another perfluoroheteroaromatic compound with similar reactivity and applications in organic synthesis.
Tetrafluoropyridazine: Known for its use as a multifunctional building block for the synthesis of macrocyclic systems.
Uniqueness of Pentafluoropyridine:
- Pentafluoropyridine is unique due to its high reactivity and versatility in undergoing nucleophilic aromatic substitution reactions. Its ability to enhance proteolytic stability in peptides and its applications in high-performance supercapacitors further distinguish it from other similar compounds .
属性
IUPAC Name |
2,3,4,5,6-pentafluoropyridine | |
---|---|---|
Source | PubChem | |
URL | https://pubchem.ncbi.nlm.nih.gov | |
Description | Data deposited in or computed by PubChem | |
InChI |
InChI=1S/C5F5N/c6-1-2(7)4(9)11-5(10)3(1)8 | |
Source | PubChem | |
URL | https://pubchem.ncbi.nlm.nih.gov | |
Description | Data deposited in or computed by PubChem | |
InChI Key |
XTGOWLIKIQLYRG-UHFFFAOYSA-N | |
Source | PubChem | |
URL | https://pubchem.ncbi.nlm.nih.gov | |
Description | Data deposited in or computed by PubChem | |
Canonical SMILES |
C1(=C(C(=NC(=C1F)F)F)F)F | |
Source | PubChem | |
URL | https://pubchem.ncbi.nlm.nih.gov | |
Description | Data deposited in or computed by PubChem | |
Molecular Formula |
C5F5N | |
Source | PubChem | |
URL | https://pubchem.ncbi.nlm.nih.gov | |
Description | Data deposited in or computed by PubChem | |
DSSTOX Substance ID |
DTXSID70220258 | |
Record name | Pentafluoropyridine | |
Source | EPA DSSTox | |
URL | https://comptox.epa.gov/dashboard/DTXSID70220258 | |
Description | DSSTox provides a high quality public chemistry resource for supporting improved predictive toxicology. | |
Molecular Weight |
169.05 g/mol | |
Source | PubChem | |
URL | https://pubchem.ncbi.nlm.nih.gov | |
Description | Data deposited in or computed by PubChem | |
CAS No. |
700-16-3 | |
Record name | Pentafluoropyridine | |
Source | CAS Common Chemistry | |
URL | https://commonchemistry.cas.org/detail?cas_rn=700-16-3 | |
Description | CAS Common Chemistry is an open community resource for accessing chemical information. Nearly 500,000 chemical substances from CAS REGISTRY cover areas of community interest, including common and frequently regulated chemicals, and those relevant to high school and undergraduate chemistry classes. This chemical information, curated by our expert scientists, is provided in alignment with our mission as a division of the American Chemical Society. | |
Explanation | The data from CAS Common Chemistry is provided under a CC-BY-NC 4.0 license, unless otherwise stated. | |
Record name | Pentafluoropyridine | |
Source | ChemIDplus | |
URL | https://pubchem.ncbi.nlm.nih.gov/substance/?source=chemidplus&sourceid=0000700163 | |
Description | ChemIDplus is a free, web search system that provides access to the structure and nomenclature authority files used for the identification of chemical substances cited in National Library of Medicine (NLM) databases, including the TOXNET system. | |
Record name | Pentafluoropyridine | |
Source | EPA DSSTox | |
URL | https://comptox.epa.gov/dashboard/DTXSID70220258 | |
Description | DSSTox provides a high quality public chemistry resource for supporting improved predictive toxicology. | |
Record name | Pentafluoropyridine | |
Source | European Chemicals Agency (ECHA) | |
URL | https://echa.europa.eu/substance-information/-/substanceinfo/100.010.765 | |
Description | The European Chemicals Agency (ECHA) is an agency of the European Union which is the driving force among regulatory authorities in implementing the EU's groundbreaking chemicals legislation for the benefit of human health and the environment as well as for innovation and competitiveness. | |
Explanation | Use of the information, documents and data from the ECHA website is subject to the terms and conditions of this Legal Notice, and subject to other binding limitations provided for under applicable law, the information, documents and data made available on the ECHA website may be reproduced, distributed and/or used, totally or in part, for non-commercial purposes provided that ECHA is acknowledged as the source: "Source: European Chemicals Agency, http://echa.europa.eu/". Such acknowledgement must be included in each copy of the material. ECHA permits and encourages organisations and individuals to create links to the ECHA website under the following cumulative conditions: Links can only be made to webpages that provide a link to the Legal Notice page. | |
Retrosynthesis Analysis
AI-Powered Synthesis Planning: Our tool employs the Template_relevance Pistachio, Template_relevance Bkms_metabolic, Template_relevance Pistachio_ringbreaker, Template_relevance Reaxys, Template_relevance Reaxys_biocatalysis model, leveraging a vast database of chemical reactions to predict feasible synthetic routes.
One-Step Synthesis Focus: Specifically designed for one-step synthesis, it provides concise and direct routes for your target compounds, streamlining the synthesis process.
Accurate Predictions: Utilizing the extensive PISTACHIO, BKMS_METABOLIC, PISTACHIO_RINGBREAKER, REAXYS, REAXYS_BIOCATALYSIS database, our tool offers high-accuracy predictions, reflecting the latest in chemical research and data.
Strategy Settings
Precursor scoring | Relevance Heuristic |
---|---|
Min. plausibility | 0.01 |
Model | Template_relevance |
Template Set | Pistachio/Bkms_metabolic/Pistachio_ringbreaker/Reaxys/Reaxys_biocatalysis |
Top-N result to add to graph | 6 |
Feasible Synthetic Routes
体外研究产品的免责声明和信息
请注意,BenchChem 上展示的所有文章和产品信息仅供信息参考。 BenchChem 上可购买的产品专为体外研究设计,这些研究在生物体外进行。体外研究,源自拉丁语 "in glass",涉及在受控实验室环境中使用细胞或组织进行的实验。重要的是要注意,这些产品没有被归类为药物或药品,他们没有得到 FDA 的批准,用于预防、治疗或治愈任何医疗状况、疾病或疾病。我们必须强调,将这些产品以任何形式引入人类或动物的身体都是法律严格禁止的。遵守这些指南对确保研究和实验的法律和道德标准的符合性至关重要。