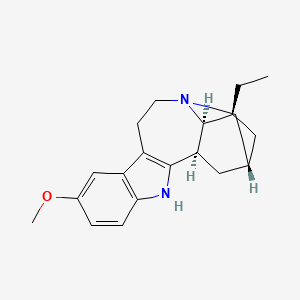
伊波加碱
描述
Ibogaine is a naturally occurring psychoactive indole alkaloid found in several plant species, most notably in the root bark of the African shrub Tabernanthe iboga. It has been traditionally used in West African spiritual ceremonies and rituals. Ibogaine is known for its potential therapeutic effects, particularly in the treatment of substance use disorders, due to its ability to reduce withdrawal symptoms and cravings .
作用机制
Target of Action
Ibogaine, an indole alkaloid derived from the African shrub Tabernanthe iboga, has a complex pharmacology, affecting many different neurotransmitter systems simultaneously . It primarily targets the opioid system, crucial in addiction and pain regulation . It is active on sigma-2 and multiple opioid receptor sites . It also interacts with acetylcholine, dopamine, and serotonin systems .
Mode of Action
Ibogaine interacts with its targets by binding to and modulating the activity of these receptors, altering the release and reuptake of neurotransmitters . This modulation is believed to contribute to the reduction of withdrawal symptoms and cravings observed in individuals undergoing ibogaine therapy for opioid addiction .
Biochemical Pathways
Ibogaine’s activity is achieved through different types of receptors, but also rapid depletion of ATP, that is followed by the induction of energy-related enzymes, as well as rise of cellular reactive oxygen species (ROS) and antioxidant enzymes activity . It is also known to modulate intracellular signaling linked to opioid receptors .
Pharmacokinetics
When given orally, ibogaine is absorbed rapidly and undergoes extensive first-pass metabolism to 12-hydroxyibogamine (noribogaine) by cytochrome P450 2D6 (CYP2D6) in the gut wall and liver . Plasma protein binding is in the range of 65–70% and consistent with its lipophilic nature, ibogaine accumulates in fat . The plasma half-life of ibogaine is in the range of 2–6 hours depending on CYP2D6 genotype, while the metabolite noribogaine is eliminated with a half-life of 24–30 hours .
Result of Action
The molecular and cellular effects of ibogaine’s action include an increased amount of the neurotransmitter in the synaptic cleft and prolongs its effect on target cells . It also leads to potential synergies by modulating multiple receptor systems .
Action Environment
The setting in which ibogaine is consumed involves aspects of the environment in which the person is located while using the substance, and it includes factors such as culture, context of use, environmental stimuli (light, music, decoration) and presence (or absence) of other people . The use of controlled settings, supported by trained professionals and equipment allowing for rigorous medical, psychiatric, and cardiac monitoring, are essential to promote the safety of patients receiving ibogaine .
科学研究应用
Ibogaine has garnered significant attention in scientific research due to its diverse applications:
Chemistry: Ibogaine and its derivatives are studied for their unique chemical structures and potential as lead compounds for drug development.
Biology: Research focuses on the biological effects of ibogaine, including its impact on neurotransmitter systems and neuroplasticity.
Medicine: Ibogaine is primarily investigated for its potential in treating substance use disorders, particularly opioid addiction. It is also explored for its effects on mood disorders and neurodegenerative diseases.
Industry: Ibogaine’s unique properties make it a subject of interest in the pharmaceutical industry for developing new therapeutic agents
生化分析
Biochemical Properties
Ibogaine plays a significant role in various biochemical reactions. It interacts with several enzymes, proteins, and other biomolecules. One of the primary enzymes involved in its metabolism is cytochrome P450 2D6 (CYP2D6), which converts ibogaine into its active metabolite, noribogaine . Ibogaine also interacts with mu-opioid receptors, serotonin transporters, and N-methyl-D-aspartate (NMDA) receptors, influencing neurotransmitter systems and receptor signaling .
Cellular Effects
Ibogaine affects various cell types and cellular processes. It influences cell function by modulating cell signaling pathways, gene expression, and cellular metabolism. For example, ibogaine has been shown to impact the expression of genes involved in neurotransmitter systems, such as dopamine and serotonin pathways . Additionally, ibogaine’s interaction with NMDA receptors affects calcium ion influx, which can influence cellular metabolism and signaling .
Molecular Mechanism
At the molecular level, ibogaine exerts its effects through several mechanisms. It binds to mu-opioid receptors, acting as an agonist, and modulates serotonin transporters, enhancing serotonin levels in the synaptic cleft . Ibogaine also inhibits NMDA receptors, reducing excitotoxicity and promoting neuroprotection . These interactions lead to changes in gene expression, particularly in genes related to neurotransmitter systems and receptor signaling .
Temporal Effects in Laboratory Settings
In laboratory settings, the effects of ibogaine change over time. Ibogaine is rapidly absorbed and metabolized into noribogaine, which has a longer half-life and sustained effects . Studies have shown that ibogaine’s effects on cellular function, such as neurotransmitter release and receptor signaling, can persist for several hours to days . The stability and degradation of ibogaine and its metabolites can influence the duration and intensity of its effects .
Dosage Effects in Animal Models
The effects of ibogaine vary with different dosages in animal models. At lower doses, ibogaine has been shown to reduce drug-seeking behavior and withdrawal symptoms in models of substance use disorders . At higher doses, ibogaine can cause toxic effects, such as neurotoxicity and cardiotoxicity . These adverse effects highlight the importance of careful dosage management in therapeutic applications .
Metabolic Pathways
Ibogaine is involved in several metabolic pathways. It is primarily metabolized by cytochrome P450 2D6 (CYP2D6) into noribogaine . This metabolic conversion is crucial for ibogaine’s therapeutic effects, as noribogaine has a longer half-life and sustained activity . Additionally, ibogaine’s metabolism can influence metabolic flux and metabolite levels, affecting its overall pharmacokinetics and pharmacodynamics .
Transport and Distribution
Ibogaine is transported and distributed within cells and tissues through various mechanisms. It is lipophilic, allowing it to accumulate in fatty tissues . Ibogaine also interacts with plasma proteins, which can influence its distribution and bioavailability . Transporters and binding proteins, such as serotonin transporters, play a role in ibogaine’s localization and accumulation within specific tissues .
Subcellular Localization
The subcellular localization of ibogaine affects its activity and function. Ibogaine can be found in various cellular compartments, including the cytoplasm and mitochondria . Post-translational modifications and targeting signals may direct ibogaine to specific organelles, influencing its interactions with biomolecules and its overall efficacy . Understanding the subcellular localization of ibogaine is essential for elucidating its mechanisms of action and therapeutic potential .
准备方法
Synthetic Routes and Reaction Conditions: Ibogaine can be synthesized through several methods. One common approach involves the semi-synthesis from voacangine, another plant alkaloid. The total synthesis of ibogaine was first described in 1956. The process typically involves multiple steps, including the formation of key intermediates and the use of specific reagents and catalysts to achieve the desired chemical transformations .
Industrial Production Methods: Industrial production of ibogaine often relies on the extraction from the root bark of Tabernanthe iboga. The extraction process involves the use of solvents to isolate the alkaloid, followed by purification steps to obtain ibogaine in its pure form. Semi-synthetic methods using voacangine as a precursor are also employed in industrial settings .
化学反应分析
Types of Reactions: Ibogaine undergoes various chemical reactions, including oxidation, reduction, and substitution reactions. These reactions are essential for modifying the structure of ibogaine and exploring its derivatives for potential therapeutic applications .
Common Reagents and Conditions:
Oxidation: Common oxidizing agents such as potassium permanganate or chromium trioxide can be used to oxidize ibogaine, leading to the formation of different oxidation products.
Reduction: Reducing agents like lithium aluminum hydride or sodium borohydride are employed to reduce ibogaine, resulting in the formation of reduced derivatives.
Substitution: Substitution reactions often involve the use of halogens or other electrophiles to introduce new functional groups into the ibogaine molecule
Major Products: The major products formed from these reactions include various ibogaine derivatives, which are studied for their potential pharmacological properties. These derivatives can exhibit different levels of psychoactivity and therapeutic potential .
相似化合物的比较
Ibogaine is part of a family of structurally similar alkaloids known as iboga alkaloids. Some of the similar compounds include:
Noribogaine: A metabolite of ibogaine with similar anti-addictive properties but reduced psychoactivity.
18-Methoxycoronaridine (18-MC): A synthetic derivative of ibogaine designed to lack psychedelic effects while retaining anti-addictive properties.
Tabernanthalog (TBG): A non-hallucinogenic analogue of ibogaine developed to treat addiction and depression with fewer side effects .
Ibogaine’s uniqueness lies in its broad spectrum of activity across multiple neurotransmitter systems and its potential to induce long-lasting changes in brain function, making it a promising candidate for treating various neurological and psychiatric disorders.
属性
CAS 编号 |
83-74-9 |
---|---|
分子式 |
C20H26N2O |
分子量 |
310.4 g/mol |
IUPAC 名称 |
(1R,15S)-17-ethyl-7-methoxy-3,13-diazapentacyclo[13.3.1.02,10.04,9.013,18]nonadeca-2(10),4(9),5,7-tetraene |
InChI |
InChI=1S/C20H26N2O/c1-3-13-8-12-9-17-19-15(6-7-22(11-12)20(13)17)16-10-14(23-2)4-5-18(16)21-19/h4-5,10,12-13,17,20-21H,3,6-9,11H2,1-2H3/t12-,13?,17-,20?/m0/s1 |
InChI 键 |
HSIBGVUMFOSJPD-WBPAKMTCSA-N |
SMILES |
CCC1CC2CC3C1N(C2)CCC4=C3NC5=C4C=C(C=C5)OC |
手性 SMILES |
CCC1C[C@H]2C[C@@H]3C1N(C2)CCC4=C3NC5=C4C=C(C=C5)OC |
规范 SMILES |
CCC1CC2CC3C1N(C2)CCC4=C3NC5=C4C=C(C=C5)OC |
熔点 |
148 °C Crystals from ethyl acetate. mp: 132 °C /Ibopamine hydrochloride/ |
83-74-9 | |
Pictograms |
Irritant |
保质期 |
Stable under recommended storage conditions. /Ibogaine hydrochloride/ |
溶解度 |
Soluble in chloroform |
同义词 |
12 Methoxyibogamine 12-Methoxyibogamine Endabuse Ibogaine NIH 10567 NIH-10567 NIH10567 |
蒸汽压力 |
3.03X10-8 mm Hg at 25 °C (est) |
产品来源 |
United States |
Retrosynthesis Analysis
AI-Powered Synthesis Planning: Our tool employs the Template_relevance Pistachio, Template_relevance Bkms_metabolic, Template_relevance Pistachio_ringbreaker, Template_relevance Reaxys, Template_relevance Reaxys_biocatalysis model, leveraging a vast database of chemical reactions to predict feasible synthetic routes.
One-Step Synthesis Focus: Specifically designed for one-step synthesis, it provides concise and direct routes for your target compounds, streamlining the synthesis process.
Accurate Predictions: Utilizing the extensive PISTACHIO, BKMS_METABOLIC, PISTACHIO_RINGBREAKER, REAXYS, REAXYS_BIOCATALYSIS database, our tool offers high-accuracy predictions, reflecting the latest in chemical research and data.
Strategy Settings
Precursor scoring | Relevance Heuristic |
---|---|
Min. plausibility | 0.01 |
Model | Template_relevance |
Template Set | Pistachio/Bkms_metabolic/Pistachio_ringbreaker/Reaxys/Reaxys_biocatalysis |
Top-N result to add to graph | 6 |
Feasible Synthetic Routes
Q1: How does ibogaine exert its effects within the body?
A1: Ibogaine interacts with various neurotransmitter systems, exhibiting micromolar affinity for N-methyl-D-aspartate (NMDA), κ-opioid, μ-opioid, and sigma-2 receptors []. It also interacts with acetylcholine, serotonin, and dopamine systems, influencing the expression of proteins like substance P, brain-derived neurotrophic factor (BDNF), c-fos, and egr-1 []. Ibogaine's primary metabolite, noribogaine, also demonstrates biological activity, significantly contributing to ibogaine's overall pharmacological profile [].
Q2: What are the key differences in the pharmacological profiles of ibogaine and noribogaine?
A2: While both ibogaine and noribogaine exhibit anti-addictive properties in animal models, they differ in potency and side-effect profile. Notably, noribogaine is a more potent indirect serotonin agonist than ibogaine, demonstrating a 10-fold higher potency in increasing extracellular serotonin levels in the nucleus accumbens of rats []. Additionally, noribogaine appears to have a reduced propensity to induce adverse effects like tremors compared to ibogaine, suggesting it might be a safer alternative for medication development [].
Q3: What role does glial cell line-derived neurotrophic factor (GDNF) play in ibogaine's actions against alcohol consumption?
A4: Research suggests that GDNF in the ventral tegmental area (VTA) is crucial in mediating ibogaine's effects on reducing alcohol consumption []. Ibogaine has been shown to increase GDNF expression in the VTA, and this increase is associated with a reduction in ethanol self-administration in rodents [, ]. The effect of ibogaine on ethanol consumption can be mimicked by injecting GDNF directly into the VTA and can be attenuated by injecting anti-GDNF antibodies, further supporting the critical role of GDNF in ibogaine's actions [].
Q4: How is ibogaine metabolized in the body?
A5: Ibogaine is primarily metabolized by the cytochrome P450 enzyme CYP2D6 to its main metabolite, noribogaine [, , ]. Noribogaine exhibits clinically relevant concentrations for an extended period, even after ibogaine is cleared from the system []. This prolonged presence of noribogaine is likely due to its slow release from fat tissue, where both ibogaine and noribogaine are sequestered [].
Q5: What is the significance of CYP2D6 polymorphisms in ibogaine metabolism?
A6: Genetic variations in the CYP2D6 enzyme can significantly influence the pharmacokinetic profiles of both ibogaine and noribogaine [, ]. Patients identified as CYP2D6 poor metabolizers exhibit a 3-fold higher blood concentration of ibogaine and a 4-fold lower concentration of noribogaine compared to extensive metabolizers []. This suggests that individuals with different CYP2D6 metabolic phenotypes may exhibit varied sensitivity to ibogaine and noribogaine, potentially influencing therapeutic outcomes and safety profiles [].
Q6: What are the implications of ibogaine and noribogaine sequestration in fat tissue?
A7: Both ibogaine and its active metabolite, noribogaine, are sequestered in fat tissue [, ]. This sequestration contributes to their complex pharmacokinetic profiles, characterized by a long elimination half-life and prolonged presence in the body even after a single dose [, ]. The slow release of ibogaine and noribogaine from fat stores could contribute to the long-lasting effects observed after a single administration [].
Q7: Are there alternative compounds being explored that might offer a safer profile than ibogaine?
A11: The development of safer analogs of ibogaine with reduced toxicity is an active area of research []. One such compound, 18-methoxycoronaridine (18-MC), has shown promise in preclinical studies. 18-MC, a synthetic derivative of ibogaine, exhibits similar anti-addictive properties in rodent models without some of ibogaine's adverse side effects, such as tremors and cerebellar damage []. Its lower affinity for NMDA and sigma-2 receptors, compared to ibogaine, is thought to contribute to its improved safety profile [].
Q8: What are the critical areas for future research on ibogaine?
A12: Future research should prioritize well-controlled clinical trials to assess the long-term safety and efficacy of ibogaine in treating substance use disorders [, , ]. Exploring personalized medicine approaches based on CYP2D6 genotyping could potentially optimize dosing and minimize adverse effects []. Further investigation into the mechanisms of action, particularly the role of different receptors and signaling pathways, is crucial for understanding ibogaine's complex pharmacology and developing safer and more effective therapies [].
体外研究产品的免责声明和信息
请注意,BenchChem 上展示的所有文章和产品信息仅供信息参考。 BenchChem 上可购买的产品专为体外研究设计,这些研究在生物体外进行。体外研究,源自拉丁语 "in glass",涉及在受控实验室环境中使用细胞或组织进行的实验。重要的是要注意,这些产品没有被归类为药物或药品,他们没有得到 FDA 的批准,用于预防、治疗或治愈任何医疗状况、疾病或疾病。我们必须强调,将这些产品以任何形式引入人类或动物的身体都是法律严格禁止的。遵守这些指南对确保研究和实验的法律和道德标准的符合性至关重要。