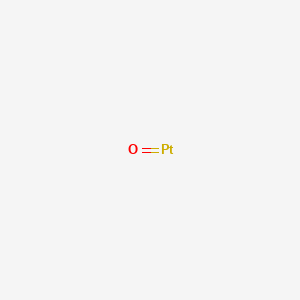
Platinum oxide
描述
Platinum oxide (PtO₂·H₂O) is a critical precursor to platinum black, a highly active catalyst for hydrogenation and oxidation reactions. It is synthesized by fusing chloroplatinic acid (H₂PtCl₆) with sodium nitrate (NaNO₃) at 450–600°C, yielding a brown granular powder that is insoluble in most acids but reducible to metallic platinum under hydrogen . The oxide’s catalytic activity depends on factors such as fusion temperature, solvent choice, and the presence of promoters (e.g., iron salts) or poisons (e.g., nitrites) . This compound-derived catalysts are widely used in organic synthesis, including reductions of nitro compounds and aldehydes .
准备方法
Solid-State Reactions and Fusion Methods
Solid-state synthesis remains a foundational approach for platinum oxide production. Platinic oxide (PtO₂) is classically prepared by fusing chloroplatinic acid (H₂PtCl₆) with sodium nitrate (NaNO₃) at elevated temperatures. This method, detailed in early organic synthesis protocols, involves heating the mixture to decompose nitrate ions, which act as oxidizing agents to convert platinum precursors into PtO₂ . The reaction proceeds as:
2\text{PtCl}6 + \text{NaNO}3 \rightarrow \text{PtO}2 + \text{NaCl} + \text{HCl} + \text{NO}_x \uparrow \quad
Similarly, calcium platinum oxides such as Ca₂Pt₃O₈ are synthesized via hydrothermal treatment of CaPt(OH)₆ at 800 K and 170 MPa, yielding crystalline structures confirmed by powder X-ray diffraction . These high-pressure conditions facilitate the formation of layered oxide frameworks, with interatomic distances refined to ±0.01 Å precision .
Wet Chemical Synthesis and pH-Mediated Precipitation
Liquid-phase methods offer scalability and reduced environmental impact compared to fusion techniques. A patented approach involves adjusting the pH of chloroplatinic acid solutions (0.25–0.4 g/mL Pt) using sodium carbonate to pH 9.5–10.5, followed by glacial acetic acid addition to pH 4–5 . This sequential pH modulation precipitates hydrated PtO₂, which is subsequently calcined at 350–400°C to yield crystalline PtO₂ with >95.7% purity . The process avoids toxic byproducts and enables gram-scale production, making it industrially viable.
Comparatively, platinic oxide nanoparticles are synthesized by depositing Pt²⁺ or Pt⁴⁺ species onto carbon nitride (C₃N₄) supports, followed by thermal reduction. X-ray photoelectron spectroscopy (XPS) reveals that Pt⁴⁺-rich precursors yield smaller nanoparticles (3–5 nm) with enhanced electrochemical activity for hydrogen evolution .
Hydrothermal and Solvothermal Techniques
Hydrothermal synthesis under confined conditions enables precise control over crystallinity and morphology. For instance, single-layer PtOₓ nanosheets (0.9 nm thickness) are exfoliated from layered potassium platinate using tert-butylammonium ions, followed by topotactic reduction to produce 0.5 nm-thick Pt nanosheets . These ultrathin structures exhibit 2.5-fold higher oxygen reduction reaction (ORR) mass activity than commercial Pt nanoparticles, attributed to maximized surface-to-volume ratios .
Electrochemical Oxidation
In situ X-ray photoelectron spectroscopy (XPS) studies reveal that platinum nanoparticles oxidize dynamically under oxygen reduction reaction (ORR) conditions. At potentials above 0.9 V (RHE), a mixed Ptδ⁺/Pt²⁺/Pt⁴⁺ surface oxide forms, transitioning to bulk PtO₂·nH₂O within seconds . Bromide adsorption from membranes suppresses oxide formation, highlighting the sensitivity of electrochemical pathways to electrolyte composition .
Thermal Decomposition and Calcination
Thermogravimetric analysis (TGA) of Ca₂Pt₃O₈ decomposition identifies four distinct steps: initial PtO₂ reduction to Pt (720°C), intermediate phase formation (855°C), and final CaO/Pt segregation (1130°C) . Similarly, calcining Pt²⁺- or Pt⁴⁺-loaded C₃N₄ at 90°C under nitrogen yields oxide nanoparticles with tunable oxidation states .
Comparative Analysis of Synthesis Methods
Structural and Mechanistic Insights
X-ray diffraction (XRD) patterns of hydrothermally synthesized Ca₂Pt₃O₈ confirm a monoclinic unit cell (a = 9.42 Å, b = 5.64 Å, c = 12.78 Å) with Pt–O bond lengths of 2.02–2.04 Å . In contrast, PtO₂ nanosheets exhibit a hexagonal lattice (d-spacing = 0.21 nm) after topotactic reduction . XPS further differentiates surface oxidation states: Pt⁴⁺ dominates in thermally decomposed samples (74.1 eV binding energy), while electrochemical oxidation produces mixed Ptδ⁺ (71.8 eV) and Pt²⁺ (72.9 eV) species .
化学反应分析
Types of Reactions: Platinum oxide undergoes various types of chemical reactions, including oxidation, reduction, and catalytic reactions.
Common Reagents and Conditions:
Oxidation: this compound can be further oxidized under specific conditions, although it is already in a high oxidation state.
Reduction: It can be reduced to platinum metal using hydrogen gas or other reducing agents.
Catalytic Reactions: this compound is widely used as a catalyst in several chemical reactions due to the high oxidation state of platinum.
Major Products Formed:
Reduction: Platinum metal (Pt)
Catalytic Reactions: Various products depending on the specific reaction being catalyzed, such as water in hydrogenation reactions.
科学研究应用
Catalytic Applications
1. Catalysis in Chemical Reactions
Platinum oxides are extensively used as catalysts in various chemical processes. They facilitate reactions by lowering the activation energy required for the reaction to proceed without being consumed in the process. Notably, platinum oxide is crucial in:
- Nitric Acid Production : Platinum-rhodium catalysts are employed in the synthesis of nitric acid, a key ingredient for fertilizers and explosives .
- Hydrogenation Reactions : this compound serves as an effective catalyst for hydrogenation processes, where it aids in the addition of hydrogen to unsaturated organic compounds .
2. Fuel Cells
this compound plays a pivotal role in fuel cell technology, particularly in proton exchange membrane fuel cells (PEMFCs). The catalyst enhances the electrochemical reactions that convert hydrogen and oxygen into water, producing electricity efficiently. Recent studies have shown that platinum oxides can improve the performance of these cells under varying humidity conditions .
Electrochemical Applications
1. Electrocatalysis
Platinum oxides are vital in electrocatalytic processes, especially for oxygen reduction reactions (ORR) which are critical for fuel cells and metal-air batteries. Research indicates that surface oxides formed during the oxidation of platinum nanoparticles significantly influence their catalytic activity .
2. Sensors
this compound-based sensors are developed for detecting gases such as carbon monoxide and hydrogen sulfide. The high surface area and catalytic properties of platinum oxides allow for sensitive detection mechanisms that are essential for environmental monitoring and safety applications .
Biomedical Applications
1. Medical Devices
Due to its biocompatibility and resistance to corrosion, this compound is used in various medical devices, including stents and pacemakers. Its radiopaque properties enhance visibility under X-ray imaging, making it suitable for cardiovascular applications .
2. Cancer Treatment
Compounds derived from platinum, including platinum oxides, have been utilized in chemotherapy drugs such as cisplatin. These compounds are effective against various types of cancer by interfering with DNA replication in cancer cells .
Summary of Key Applications
Application Area | Specific Uses |
---|---|
Catalysis | Nitric acid production, hydrogenation reactions |
Fuel Cells | Proton exchange membrane fuel cells |
Electrocatalysis | Oxygen reduction reactions |
Sensors | Gas detection (e.g., carbon monoxide) |
Biomedical Devices | Stents, pacemakers |
Cancer Treatment | Chemotherapy drugs |
Case Study 1: Fuel Cell Performance Enhancement
A study published in 2024 identified a two-dimensional crystalline form of this compound that exhibits remarkable thermal stability at high temperatures (up to 1200 K). This innovation could lead to more efficient fuel cells capable of operating under extreme conditions, thus broadening their application range .
Case Study 2: Electrocatalytic Efficiency
Research conducted on the oxidation of platinum nanoparticles revealed that the formation of surface oxides significantly affects their catalytic behavior during electrochemical processes. This study highlighted how optimizing the environment around these nanoparticles can enhance their performance in fuel cell applications .
作用机制
The mechanism by which platinum oxide exerts its effects is primarily through its catalytic properties. In catalytic reactions, this compound facilitates the conversion of reactants to products by providing an alternative reaction pathway with a lower activation energy. This is achieved through the adsorption of reactants onto the this compound surface, where they undergo chemical transformations before being released as products .
Molecular Targets and Pathways:
- In fuel cells, this compound catalyzes the oxygen reduction reaction, which is crucial for the generation of electricity.
- In biomedical applications, this compound nanoparticles interact with cellular components, potentially leading to therapeutic effects such as the generation of reactive oxygen species that can target cancer cells .
相似化合物的比较
Palladium Oxide (PdO)
- Preparation & Structure: Palladium oxide is synthesized by fusing palladous chloride (PdCl₂) with NaNO₃ at 600°C, forming PdO with a tetragonal crystal structure .
- Catalytic Activity : PdO exhibits comparable hydrogenation activity to PtO₂ in some cases (e.g., nitro group reductions) but is less effective for substrates requiring strong metal-adsorption sites (e.g., aromatic rings) .
- Stability : PdO-based catalysts are more prone to sintering than PtO₂-derived systems, particularly on silica or alumina supports .
Ruthenium Dioxide (RuO₂) and Iridium Dioxide (IrO₂)
- Electrocatalytic Performance: RuO₂ and IrO₂ are benchmark catalysts for the oxygen evolution reaction (OER) in water electrolysis, outperforming PtO₂ in acidic conditions. For example, Ir₀.₅Ru₀.₅O₂ achieves a current density of 10 mA/cm² at an overpotential of 250 mV, while PtO₂ requires >400 mV .
- Mechanistic Differences : RuO₂ and IrO₂ stabilize oxygen intermediates (e.g., *OOH) more effectively than PtO₂ due to their lower d-band centers .
Iron Oxide (Fe₂O₃) and Tin Dioxide (SnO₂) Composites
- Synergistic Effects: Platinum nanoparticles supported on Fe₂O₃ or SnO₂ exhibit enhanced CO oxidation activity. For instance, Pt/Fe₂O₃ shows a 40% higher turnover frequency (TOF) for CO oxidation than Pt/Al₂O₃ due to metal-support interactions that stabilize active Pt⁰ sites .
- Gas Sensing vs. Catalysis: Pt/SnO₂ composites excel in CO sensing but underperform in catalysis due to conflicting requirements (reversible surface changes for sensing vs. irreversible oxidation for catalysis) .
Structural and Functional Data
Research Findings and Trends
- Support Effects : Platinum oxide on high-entropy aluminate spinels retains 90% dispersion after thermal aging (vs. 50% on alumina) due to strong Pt-support anchoring .
- Alternative Materials : Graphene-MoS₂ hybrids achieve 95% of PtO₂’s efficiency in dye-sensitized solar cells (DSSCs), suggesting cost-effective alternatives for specific uses .
常见问题
Basic Research Questions
Q. What are the standard laboratory methods for synthesizing platinum oxide, and how do procedural variations impact yield and purity?
The Adams method involves fusing chloroplatinic acid (H₂PtCl₆) with sodium nitrate (NaNO₃) at 450–600°C. Yields are near-quantitative (>95%) at optimal temperatures, while lower temperatures (<450°C) reduce yield due to incomplete fusion, and higher temperatures (>600°C) risk spattering. Impurities in chloroplatinic acid (e.g., organic residues) require purification via ammonium chloroplatinate precipitation to ensure catalytic activity .
Q. How is this compound characterized to confirm its chemical composition and structural integrity?
Elemental analysis via flame atomic absorption (AA) after aqua regia digestion confirms platinum content (92.41% Pt in PtO₂). X-ray diffraction (XRD) identifies crystalline phases, while gravimetric analysis verifies stoichiometry (e.g., PtO₂·H₂O). Residual sodium nitrate is detected through water extraction and conductivity testing .
Q. What safety protocols are critical when handling this compound in laboratory settings?
Use N95 masks, nitrile gloves, and eye protection to avoid inhalation or contact. Test filtrates for residual platinum using stannous chloride (SnCl₂): a yellow color indicates Pt²⁺/Pt⁴⁺. Avoid storage with alcohols or strong oxidizers to prevent reactive decomposition .
Q. What methods are effective for recovering and reactivating spent this compound catalysts?
Dissolve spent catalysts in aqua regia, filter, and reprecipitate via NaNO₃ fusion. Reactivate by reducing with hydrogen (H₂) in ethanol, but repeated cycles require Wichers’ purification (e.g., sodium nitrate fusion followed by HCl washing) to remove accumulated poisons like sulfur compounds .
Advanced Research Questions
Q. What experimental factors influence the reduction lag time of this compound to platinum black during catalytic hydrogenation?
Lag time (seconds to 15 minutes) depends on solvent (alcohols reduce lag vs. acetic acid), substrate presence (aldehydes accelerate reduction), and promoters (e.g., Fe³⁺) or poisons (e.g., NaNO₂). For example, benzaldehyde reduces lag to <1 minute by promoting finer Pt black dispersion .
Q. How do hybrid support structures like graphene-metal oxide composites enhance this compound catalysts' electrochemical performance?
Triple-junction structures (e.g., Pt/indium tin oxide (ITO)/graphene) stabilize nanoparticles via "paperweight" effects, reducing agglomeration. In oxygen reduction reactions (ORR), these systems show 3x higher stability over 100 cycles compared to carbon-alone supports .
Q. How does the incorporation of rhodium into this compound matrices affect catalytic selectivity in hydrogenation reactions?
Rh-Pt oxide (prepared via NaNO₃ fusion at 460–480°C) enhances selectivity for aromatic ketone reductions (e.g., acetophenone to phenylethanol) due to Rh-induced electronic modulation. Activity correlates with Rh:Pt ratios (optimal 3:1), though excess Rh (>50%) poisons active sites .
Q. How do pre-treatment atmospheres (e.g., H₂/Ar) influence the stability of carbon-supported this compound electrocatalysts?
H₂/Ar annealing at 300°C reduces surface oxides, strengthening Pt-C interactions. This pre-treatment improves stability in proton-exchange membrane fuel cells (PEMFCs), with <10% performance loss after 200 hours vs. 40% loss in untreated catalysts .
Q. Methodological Tables
属性
IUPAC Name |
oxoplatinum | |
---|---|---|
Source | PubChem | |
URL | https://pubchem.ncbi.nlm.nih.gov | |
Description | Data deposited in or computed by PubChem | |
InChI |
InChI=1S/O.Pt | |
Source | PubChem | |
URL | https://pubchem.ncbi.nlm.nih.gov | |
Description | Data deposited in or computed by PubChem | |
InChI Key |
MUMZUERVLWJKNR-UHFFFAOYSA-N | |
Source | PubChem | |
URL | https://pubchem.ncbi.nlm.nih.gov | |
Description | Data deposited in or computed by PubChem | |
Canonical SMILES |
O=[Pt] | |
Source | PubChem | |
URL | https://pubchem.ncbi.nlm.nih.gov | |
Description | Data deposited in or computed by PubChem | |
Molecular Formula |
OPt | |
Source | PubChem | |
URL | https://pubchem.ncbi.nlm.nih.gov | |
Description | Data deposited in or computed by PubChem | |
DSSTOX Substance ID |
DTXSID1065188 | |
Record name | Platinum oxide (PtO) | |
Source | EPA DSSTox | |
URL | https://comptox.epa.gov/dashboard/DTXSID1065188 | |
Description | DSSTox provides a high quality public chemistry resource for supporting improved predictive toxicology. | |
Molecular Weight |
211.08 g/mol | |
Source | PubChem | |
URL | https://pubchem.ncbi.nlm.nih.gov | |
Description | Data deposited in or computed by PubChem | |
CAS No. |
11129-89-8, 12035-82-4 | |
Record name | Platinum oxide | |
Source | CAS Common Chemistry | |
URL | https://commonchemistry.cas.org/detail?cas_rn=11129-89-8 | |
Description | CAS Common Chemistry is an open community resource for accessing chemical information. Nearly 500,000 chemical substances from CAS REGISTRY cover areas of community interest, including common and frequently regulated chemicals, and those relevant to high school and undergraduate chemistry classes. This chemical information, curated by our expert scientists, is provided in alignment with our mission as a division of the American Chemical Society. | |
Explanation | The data from CAS Common Chemistry is provided under a CC-BY-NC 4.0 license, unless otherwise stated. | |
Record name | Platinum oxide (PtO) | |
Source | CAS Common Chemistry | |
URL | https://commonchemistry.cas.org/detail?cas_rn=12035-82-4 | |
Description | CAS Common Chemistry is an open community resource for accessing chemical information. Nearly 500,000 chemical substances from CAS REGISTRY cover areas of community interest, including common and frequently regulated chemicals, and those relevant to high school and undergraduate chemistry classes. This chemical information, curated by our expert scientists, is provided in alignment with our mission as a division of the American Chemical Society. | |
Explanation | The data from CAS Common Chemistry is provided under a CC-BY-NC 4.0 license, unless otherwise stated. | |
Record name | Platinum oxide (PtO) | |
Source | ChemIDplus | |
URL | https://pubchem.ncbi.nlm.nih.gov/substance/?source=chemidplus&sourceid=0012035824 | |
Description | ChemIDplus is a free, web search system that provides access to the structure and nomenclature authority files used for the identification of chemical substances cited in National Library of Medicine (NLM) databases, including the TOXNET system. | |
Record name | Platinum oxide | |
Source | DTP/NCI | |
URL | https://dtp.cancer.gov/dtpstandard/servlet/dwindex?searchtype=NSC&outputformat=html&searchlist=128165 | |
Description | The NCI Development Therapeutics Program (DTP) provides services and resources to the academic and private-sector research communities worldwide to facilitate the discovery and development of new cancer therapeutic agents. | |
Explanation | Unless otherwise indicated, all text within NCI products is free of copyright and may be reused without our permission. Credit the National Cancer Institute as the source. | |
Record name | Platinum oxide (PtO) | |
Source | EPA Chemicals under the TSCA | |
URL | https://www.epa.gov/chemicals-under-tsca | |
Description | EPA Chemicals under the Toxic Substances Control Act (TSCA) collection contains information on chemicals and their regulations under TSCA, including non-confidential content from the TSCA Chemical Substance Inventory and Chemical Data Reporting. | |
Record name | Platinum oxide (PtO) | |
Source | EPA DSSTox | |
URL | https://comptox.epa.gov/dashboard/DTXSID1065188 | |
Description | DSSTox provides a high quality public chemistry resource for supporting improved predictive toxicology. | |
Record name | Platinum oxide | |
Source | European Chemicals Agency (ECHA) | |
URL | https://echa.europa.eu/substance-information/-/substanceinfo/100.031.652 | |
Description | The European Chemicals Agency (ECHA) is an agency of the European Union which is the driving force among regulatory authorities in implementing the EU's groundbreaking chemicals legislation for the benefit of human health and the environment as well as for innovation and competitiveness. | |
Explanation | Use of the information, documents and data from the ECHA website is subject to the terms and conditions of this Legal Notice, and subject to other binding limitations provided for under applicable law, the information, documents and data made available on the ECHA website may be reproduced, distributed and/or used, totally or in part, for non-commercial purposes provided that ECHA is acknowledged as the source: "Source: European Chemicals Agency, http://echa.europa.eu/". Such acknowledgement must be included in each copy of the material. ECHA permits and encourages organisations and individuals to create links to the ECHA website under the following cumulative conditions: Links can only be made to webpages that provide a link to the Legal Notice page. | |
体外研究产品的免责声明和信息
请注意,BenchChem 上展示的所有文章和产品信息仅供信息参考。 BenchChem 上可购买的产品专为体外研究设计,这些研究在生物体外进行。体外研究,源自拉丁语 "in glass",涉及在受控实验室环境中使用细胞或组织进行的实验。重要的是要注意,这些产品没有被归类为药物或药品,他们没有得到 FDA 的批准,用于预防、治疗或治愈任何医疗状况、疾病或疾病。我们必须强调,将这些产品以任何形式引入人类或动物的身体都是法律严格禁止的。遵守这些指南对确保研究和实验的法律和道德标准的符合性至关重要。