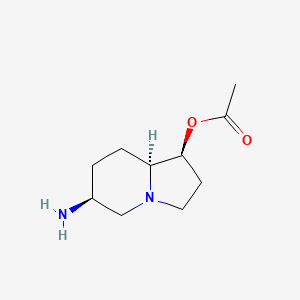
斯拉夫拉明
描述
Slaframine is a bicyclic alkaloid mycotoxin produced by the fungus Rhizoctonia leguminicola, which is a common pathogen of red clover (Trifolium pratense) . This compound is known for causing excessive salivation, commonly referred to as “slobbers,” in various animals . Slaframine has the molecular formula C₁₀H₁₈N₂O₂ and is characterized by its unique structure, which includes an indolizidine ring system .
科学研究应用
Slaframine has several scientific research applications, including:
作用机制
安全和危害
Slaframine causes a condition known as “slobbers syndrome” in livestock that have ingested contaminated feed . It is advised to avoid dust formation, breathing mist, gas or vapours, and contacting with skin and eye. Use of personal protective equipment and chemical impermeable gloves is recommended .
未来方向
The timing of gene expression was similar in cultured fungus and inoculated leaves and agreed with the proposed biosynthetic pathway . Substantially more swainsonine was produced than slaframine during time course studies . Future research could focus on further understanding the biosynthesis of Slaframine and its effects on different animal species.
准备方法
Slaframine is typically produced by the fungus Rhizoctonia leguminicola under wet and humid conditions . The industrial production of slaframine involves the cultivation of this fungus on suitable substrates, such as red clover, under controlled environmental conditions to maximize the yield of the mycotoxin . The biosynthesis of slaframine in the fungus involves several enzymatic steps, including the conversion of pipecolic acid to hydroxyindolizidine .
化学反应分析
Slaframine undergoes various chemical reactions, including:
Oxidation: Slaframine can be oxidized to form its corresponding N-oxide derivative.
Reduction: Reduction of slaframine can lead to the formation of its reduced analogs.
Substitution: Slaframine can undergo nucleophilic substitution reactions, particularly at the acetate ester group.
Common reagents used in these reactions include oxidizing agents like hydrogen peroxide for oxidation, reducing agents like sodium borohydride for reduction, and nucleophiles like amines for substitution reactions . The major products formed from these reactions include N-oxide derivatives, reduced analogs, and substituted indolizidine compounds .
相似化合物的比较
Slaframine belongs to the class of indolizidine alkaloids, which includes several other compounds with similar structures and biological activities . Some of the similar compounds include:
Swainsonine: Another mycotoxin produced by Rhizoctonia leguminicola, known for causing locoism in livestock.
Castanospermine: An indolizidine alkaloid with anti-cancer and anti-HIV properties.
Pumiliotoxin A: An indolizidine alkaloid found in the skin of poison-dart frogs, known for its effects on muscle contraction.
Slaframine is unique among these compounds due to its specific parasympathomimetic activity, which primarily affects exocrine gland function .
属性
IUPAC Name |
[(1S,6S,8aS)-6-amino-1,2,3,5,6,7,8,8a-octahydroindolizin-1-yl] acetate | |
---|---|---|
Source | PubChem | |
URL | https://pubchem.ncbi.nlm.nih.gov | |
Description | Data deposited in or computed by PubChem | |
InChI |
InChI=1S/C10H18N2O2/c1-7(13)14-10-4-5-12-6-8(11)2-3-9(10)12/h8-10H,2-6,11H2,1H3/t8-,9-,10-/m0/s1 | |
Source | PubChem | |
URL | https://pubchem.ncbi.nlm.nih.gov | |
Description | Data deposited in or computed by PubChem | |
InChI Key |
YYIUHLPAZILPSG-GUBZILKMSA-N | |
Source | PubChem | |
URL | https://pubchem.ncbi.nlm.nih.gov | |
Description | Data deposited in or computed by PubChem | |
Canonical SMILES |
CC(=O)OC1CCN2C1CCC(C2)N | |
Source | PubChem | |
URL | https://pubchem.ncbi.nlm.nih.gov | |
Description | Data deposited in or computed by PubChem | |
Isomeric SMILES |
CC(=O)O[C@H]1CCN2[C@H]1CC[C@@H](C2)N | |
Source | PubChem | |
URL | https://pubchem.ncbi.nlm.nih.gov | |
Description | Data deposited in or computed by PubChem | |
Molecular Formula |
C10H18N2O2 | |
Source | PubChem | |
URL | https://pubchem.ncbi.nlm.nih.gov | |
Description | Data deposited in or computed by PubChem | |
DSSTOX Substance ID |
DTXSID50942108 | |
Record name | (1S,6S,8aS)-1-Acetoxy-6-aminooctahydroindolizine | |
Source | EPA DSSTox | |
URL | https://comptox.epa.gov/dashboard/DTXSID50942108 | |
Description | DSSTox provides a high quality public chemistry resource for supporting improved predictive toxicology. | |
Molecular Weight |
198.26 g/mol | |
Source | PubChem | |
URL | https://pubchem.ncbi.nlm.nih.gov | |
Description | Data deposited in or computed by PubChem | |
CAS No. |
20084-93-9 | |
Record name | (-)-Slaframine | |
Source | CAS Common Chemistry | |
URL | https://commonchemistry.cas.org/detail?cas_rn=20084-93-9 | |
Description | CAS Common Chemistry is an open community resource for accessing chemical information. Nearly 500,000 chemical substances from CAS REGISTRY cover areas of community interest, including common and frequently regulated chemicals, and those relevant to high school and undergraduate chemistry classes. This chemical information, curated by our expert scientists, is provided in alignment with our mission as a division of the American Chemical Society. | |
Explanation | The data from CAS Common Chemistry is provided under a CC-BY-NC 4.0 license, unless otherwise stated. | |
Record name | Slaframine | |
Source | ChemIDplus | |
URL | https://pubchem.ncbi.nlm.nih.gov/substance/?source=chemidplus&sourceid=0020084939 | |
Description | ChemIDplus is a free, web search system that provides access to the structure and nomenclature authority files used for the identification of chemical substances cited in National Library of Medicine (NLM) databases, including the TOXNET system. | |
Record name | (1S,6S,8aS)-1-Acetoxy-6-aminooctahydroindolizine | |
Source | EPA DSSTox | |
URL | https://comptox.epa.gov/dashboard/DTXSID50942108 | |
Description | DSSTox provides a high quality public chemistry resource for supporting improved predictive toxicology. | |
Record name | SLAFRAMINE | |
Source | FDA Global Substance Registration System (GSRS) | |
URL | https://gsrs.ncats.nih.gov/ginas/app/beta/substances/51H2386GWI | |
Description | The FDA Global Substance Registration System (GSRS) enables the efficient and accurate exchange of information on what substances are in regulated products. Instead of relying on names, which vary across regulatory domains, countries, and regions, the GSRS knowledge base makes it possible for substances to be defined by standardized, scientific descriptions. | |
Explanation | Unless otherwise noted, the contents of the FDA website (www.fda.gov), both text and graphics, are not copyrighted. They are in the public domain and may be republished, reprinted and otherwise used freely by anyone without the need to obtain permission from FDA. Credit to the U.S. Food and Drug Administration as the source is appreciated but not required. | |
Retrosynthesis Analysis
AI-Powered Synthesis Planning: Our tool employs the Template_relevance Pistachio, Template_relevance Bkms_metabolic, Template_relevance Pistachio_ringbreaker, Template_relevance Reaxys, Template_relevance Reaxys_biocatalysis model, leveraging a vast database of chemical reactions to predict feasible synthetic routes.
One-Step Synthesis Focus: Specifically designed for one-step synthesis, it provides concise and direct routes for your target compounds, streamlining the synthesis process.
Accurate Predictions: Utilizing the extensive PISTACHIO, BKMS_METABOLIC, PISTACHIO_RINGBREAKER, REAXYS, REAXYS_BIOCATALYSIS database, our tool offers high-accuracy predictions, reflecting the latest in chemical research and data.
Strategy Settings
Precursor scoring | Relevance Heuristic |
---|---|
Min. plausibility | 0.01 |
Model | Template_relevance |
Template Set | Pistachio/Bkms_metabolic/Pistachio_ringbreaker/Reaxys/Reaxys_biocatalysis |
Top-N result to add to graph | 6 |
Feasible Synthetic Routes
体外研究产品的免责声明和信息
请注意,BenchChem 上展示的所有文章和产品信息仅供信息参考。 BenchChem 上可购买的产品专为体外研究设计,这些研究在生物体外进行。体外研究,源自拉丁语 "in glass",涉及在受控实验室环境中使用细胞或组织进行的实验。重要的是要注意,这些产品没有被归类为药物或药品,他们没有得到 FDA 的批准,用于预防、治疗或治愈任何医疗状况、疾病或疾病。我们必须强调,将这些产品以任何形式引入人类或动物的身体都是法律严格禁止的。遵守这些指南对确保研究和实验的法律和道德标准的符合性至关重要。