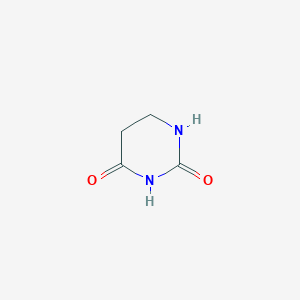
二氢尿嘧啶
描述
二氢尿嘧啶是一种属于嘧啶酮类别的有机化合物。它是尿嘧啶(RNA中的一种核碱基)分解代谢过程中的中间产物。
科学研究应用
二氢尿嘧啶有几个科学研究应用。 它是尿嘧啶分解代谢过程中的关键中间体,用于合成新型二氢尿嘧啶 。在临床化学中,二氢尿嘧啶是嘧啶代谢的代谢产物,存在于尿液、血浆和唾液中。 它的定量分析可以帮助识别氟嘧啶诱导的毒性和嘧啶代谢遗传性疾病的风险较高患者 。 此外,二氢尿嘧啶用于开发用于无创筛查的高效液相色谱方法 。
作用机制
二氢尿嘧啶的作用机制包括其作为尿嘧啶分解代谢过程中的中间体的作用。 它被酶二氢尿嘧啶氧化酶转化为尿嘧啶,氧气作为反应物,过氧化氢作为副产物 。 这个过程是嘧啶代谢途径的一部分,对核苷酸的分解和循环至关重要 。
生化分析
Biochemical Properties
Dihydrouracil plays a pivotal role in biochemical reactions, particularly in the catabolic pathway of uracil. The enzyme dihydropyrimidine dehydrogenase catalyzes the reduction of uracil to dihydrouracil. This enzyme is a flavoprotein that utilizes nicotinamide adenine dinucleotide phosphate as a cofactor. The interaction between dihydropyrimidine dehydrogenase and dihydrouracil is crucial for the proper degradation of pyrimidines, ensuring the balance of nucleotide pools within the cell .
Additionally, dihydrouracil interacts with other biomolecules, such as dihydropyrimidinase and beta-ureidopropionase, which further degrade dihydrouracil into beta-alanine, ammonia, and carbon dioxide. These interactions highlight the importance of dihydrouracil in maintaining cellular homeostasis and facilitating the recycling of nitrogenous bases .
Cellular Effects
Dihydrouracil influences various cellular processes, including cell signaling pathways, gene expression, and cellular metabolism. In particular, the accumulation of dihydrouracil can affect the regulation of pyrimidine biosynthesis and degradation. This compound has been shown to modulate the activity of enzymes involved in nucleotide metabolism, thereby impacting the overall nucleotide balance within the cell .
Furthermore, dihydrouracil can influence gene expression by affecting the availability of pyrimidine nucleotides required for RNA synthesis. This, in turn, can alter the expression of genes involved in cell growth, differentiation, and apoptosis. The impact of dihydrouracil on cellular metabolism is also significant, as it plays a role in the recycling of nitrogenous bases and the production of energy through the degradation of pyrimidines .
Molecular Mechanism
The molecular mechanism of dihydrouracil involves its interaction with specific enzymes and biomolecules. Dihydrouracil binds to the active site of dihydropyrimidine dehydrogenase, where it undergoes reduction to form dihydrouracil. This binding interaction is facilitated by the presence of nicotinamide adenine dinucleotide phosphate, which acts as a cofactor in the reduction reaction .
In addition to enzyme binding, dihydrouracil can also influence the activity of other proteins involved in pyrimidine metabolism. For example, dihydrouracil can inhibit the activity of thymidylate synthase, an enzyme responsible for the synthesis of thymidine monophosphate from deoxyuridine monophosphate. This inhibition can lead to a decrease in the availability of thymidine monophosphate, thereby affecting DNA synthesis and cell proliferation .
Temporal Effects in Laboratory Settings
In laboratory settings, the effects of dihydrouracil can change over time due to its stability and degradation. Dihydrouracil is relatively stable under physiological conditions, but it can undergo degradation in the presence of specific enzymes, such as dihydropyrimidinase and beta-ureidopropionase . These enzymes further break down dihydrouracil into beta-alanine, ammonia, and carbon dioxide, which can have long-term effects on cellular function.
Studies have shown that the accumulation of dihydrouracil over time can lead to alterations in cellular metabolism and gene expression. In vitro and in vivo studies have demonstrated that prolonged exposure to dihydrouracil can result in changes in cell growth, differentiation, and apoptosis . These temporal effects highlight the importance of monitoring dihydrouracil levels in laboratory experiments to ensure accurate and reliable results.
Dosage Effects in Animal Models
The effects of dihydrouracil can vary with different dosages in animal models. At low doses, dihydrouracil can support the normal degradation of pyrimidines and maintain cellular homeostasis. At high doses, dihydrouracil can exhibit toxic effects, leading to disruptions in nucleotide metabolism and cellular function .
Threshold effects have been observed in studies where high doses of dihydrouracil resulted in the inhibition of key enzymes involved in pyrimidine metabolism, such as thymidylate synthase. This inhibition can lead to a decrease in DNA synthesis and cell proliferation, ultimately causing adverse effects on tissue growth and development . It is crucial to determine the appropriate dosage of dihydrouracil in animal models to avoid potential toxic effects and ensure accurate experimental outcomes.
Metabolic Pathways
Dihydrouracil is involved in several metabolic pathways, primarily in the catabolism of pyrimidines. The reduction of uracil to dihydrouracil by dihydropyrimidine dehydrogenase is the first step in the degradation pathway. This is followed by the further breakdown of dihydrouracil by dihydropyrimidinase and beta-ureidopropionase into beta-alanine, ammonia, and carbon dioxide .
These metabolic pathways are essential for the recycling of nitrogenous bases and the production of energy. Dihydrouracil interacts with various enzymes and cofactors, such as nicotinamide adenine dinucleotide phosphate, to facilitate these reactions. The proper functioning of these pathways is crucial for maintaining cellular homeostasis and supporting normal cellular processes .
Transport and Distribution
Dihydrouracil is transported and distributed within cells and tissues through specific transporters and binding proteins. These transporters facilitate the movement of dihydrouracil across cellular membranes, ensuring its availability for metabolic reactions. Additionally, binding proteins can interact with dihydrouracil to regulate its localization and accumulation within cells .
The distribution of dihydrouracil within tissues can also be influenced by its interactions with other biomolecules. For example, dihydrouracil can bind to ribonucleic acid molecules, affecting their stability and function. These interactions highlight the importance of understanding the transport and distribution mechanisms of dihydrouracil to elucidate its role in cellular processes .
Subcellular Localization
The subcellular localization of dihydrouracil is primarily within the cytoplasm, where it participates in the catabolism of pyrimidines. Dihydrouracil can also be found in other cellular compartments, such as the nucleus, where it may influence gene expression and DNA synthesis .
Targeting signals and post-translational modifications can direct dihydrouracil to specific compartments or organelles within the cell. These modifications can affect the activity and function of dihydrouracil, highlighting the importance of its subcellular localization in regulating cellular processes .
准备方法
化学反应分析
二氢尿嘧啶经历了几种类型的化学反应,包括氧化和水解。 例如,二氢尿嘧啶可以使用二氢尿嘧啶氧化酶将氧气作为反应物,过氧化氢作为副产物氧化成尿嘧啶 。 这些反应中常用的试剂包括 3-氯过氧苯甲酸和甲酸 。 这些反应形成的主要产物是尿嘧啶和过氧化氢 。
相似化合物的比较
二氢尿嘧啶与其他嘧啶酮类似,例如尿嘧啶和二氢尿苷。 它在尿嘧啶分解代谢过程中的中间产物作用中是独一无二的 。 与存在于 RNA 中的核碱基尿嘧啶不同,二氢尿嘧啶不掺入核酸,而是参与其分解 。 另一方面,二氢尿苷是在转移 RNA 中发现的一种修饰的核苷 。
属性
IUPAC Name |
1,3-diazinane-2,4-dione | |
---|---|---|
Source | PubChem | |
URL | https://pubchem.ncbi.nlm.nih.gov | |
Description | Data deposited in or computed by PubChem | |
InChI |
InChI=1S/C4H6N2O2/c7-3-1-2-5-4(8)6-3/h1-2H2,(H2,5,6,7,8) | |
Source | PubChem | |
URL | https://pubchem.ncbi.nlm.nih.gov | |
Description | Data deposited in or computed by PubChem | |
InChI Key |
OIVLITBTBDPEFK-UHFFFAOYSA-N | |
Source | PubChem | |
URL | https://pubchem.ncbi.nlm.nih.gov | |
Description | Data deposited in or computed by PubChem | |
Canonical SMILES |
C1CNC(=O)NC1=O | |
Source | PubChem | |
URL | https://pubchem.ncbi.nlm.nih.gov | |
Description | Data deposited in or computed by PubChem | |
Molecular Formula |
C4H6N2O2 | |
Source | PubChem | |
URL | https://pubchem.ncbi.nlm.nih.gov | |
Description | Data deposited in or computed by PubChem | |
DSSTOX Substance ID |
DTXSID7060122 | |
Record name | 2,4(1H,3H)-Pyrimidinedione, dihydro- | |
Source | EPA DSSTox | |
URL | https://comptox.epa.gov/dashboard/DTXSID7060122 | |
Description | DSSTox provides a high quality public chemistry resource for supporting improved predictive toxicology. | |
Molecular Weight |
114.10 g/mol | |
Source | PubChem | |
URL | https://pubchem.ncbi.nlm.nih.gov | |
Description | Data deposited in or computed by PubChem | |
Physical Description |
Solid | |
Record name | Dihydrouracil | |
Source | Human Metabolome Database (HMDB) | |
URL | http://www.hmdb.ca/metabolites/HMDB0000076 | |
Description | The Human Metabolome Database (HMDB) is a freely available electronic database containing detailed information about small molecule metabolites found in the human body. | |
Explanation | HMDB is offered to the public as a freely available resource. Use and re-distribution of the data, in whole or in part, for commercial purposes requires explicit permission of the authors and explicit acknowledgment of the source material (HMDB) and the original publication (see the HMDB citing page). We ask that users who download significant portions of the database cite the HMDB paper in any resulting publications. | |
CAS No. |
504-07-4 | |
Record name | Dihydrouracil | |
Source | CAS Common Chemistry | |
URL | https://commonchemistry.cas.org/detail?cas_rn=504-07-4 | |
Description | CAS Common Chemistry is an open community resource for accessing chemical information. Nearly 500,000 chemical substances from CAS REGISTRY cover areas of community interest, including common and frequently regulated chemicals, and those relevant to high school and undergraduate chemistry classes. This chemical information, curated by our expert scientists, is provided in alignment with our mission as a division of the American Chemical Society. | |
Explanation | The data from CAS Common Chemistry is provided under a CC-BY-NC 4.0 license, unless otherwise stated. | |
Record name | Dihydrouracil | |
Source | ChemIDplus | |
URL | https://pubchem.ncbi.nlm.nih.gov/substance/?source=chemidplus&sourceid=0000504074 | |
Description | ChemIDplus is a free, web search system that provides access to the structure and nomenclature authority files used for the identification of chemical substances cited in National Library of Medicine (NLM) databases, including the TOXNET system. | |
Record name | Dihydrouracil | |
Source | DrugBank | |
URL | https://www.drugbank.ca/drugs/DB01849 | |
Description | The DrugBank database is a unique bioinformatics and cheminformatics resource that combines detailed drug (i.e. chemical, pharmacological and pharmaceutical) data with comprehensive drug target (i.e. sequence, structure, and pathway) information. | |
Explanation | Creative Common's Attribution-NonCommercial 4.0 International License (http://creativecommons.org/licenses/by-nc/4.0/legalcode) | |
Record name | dihydrouracil | |
Source | DTP/NCI | |
URL | https://dtp.cancer.gov/dtpstandard/servlet/dwindex?searchtype=NSC&outputformat=html&searchlist=11867 | |
Description | The NCI Development Therapeutics Program (DTP) provides services and resources to the academic and private-sector research communities worldwide to facilitate the discovery and development of new cancer therapeutic agents. | |
Explanation | Unless otherwise indicated, all text within NCI products is free of copyright and may be reused without our permission. Credit the National Cancer Institute as the source. | |
Record name | 2,4(1H,3H)-Pyrimidinedione, dihydro- | |
Source | EPA Chemicals under the TSCA | |
URL | https://www.epa.gov/chemicals-under-tsca | |
Description | EPA Chemicals under the Toxic Substances Control Act (TSCA) collection contains information on chemicals and their regulations under TSCA, including non-confidential content from the TSCA Chemical Substance Inventory and Chemical Data Reporting. | |
Record name | 2,4(1H,3H)-Pyrimidinedione, dihydro- | |
Source | EPA DSSTox | |
URL | https://comptox.epa.gov/dashboard/DTXSID7060122 | |
Description | DSSTox provides a high quality public chemistry resource for supporting improved predictive toxicology. | |
Record name | Dihydrouracil | |
Source | European Chemicals Agency (ECHA) | |
URL | https://echa.europa.eu/substance-information/-/substanceinfo/100.007.257 | |
Description | The European Chemicals Agency (ECHA) is an agency of the European Union which is the driving force among regulatory authorities in implementing the EU's groundbreaking chemicals legislation for the benefit of human health and the environment as well as for innovation and competitiveness. | |
Explanation | Use of the information, documents and data from the ECHA website is subject to the terms and conditions of this Legal Notice, and subject to other binding limitations provided for under applicable law, the information, documents and data made available on the ECHA website may be reproduced, distributed and/or used, totally or in part, for non-commercial purposes provided that ECHA is acknowledged as the source: "Source: European Chemicals Agency, http://echa.europa.eu/". Such acknowledgement must be included in each copy of the material. ECHA permits and encourages organisations and individuals to create links to the ECHA website under the following cumulative conditions: Links can only be made to webpages that provide a link to the Legal Notice page. | |
Record name | DIHYDROURACIL | |
Source | FDA Global Substance Registration System (GSRS) | |
URL | https://gsrs.ncats.nih.gov/ginas/app/beta/substances/016FR52RU5 | |
Description | The FDA Global Substance Registration System (GSRS) enables the efficient and accurate exchange of information on what substances are in regulated products. Instead of relying on names, which vary across regulatory domains, countries, and regions, the GSRS knowledge base makes it possible for substances to be defined by standardized, scientific descriptions. | |
Explanation | Unless otherwise noted, the contents of the FDA website (www.fda.gov), both text and graphics, are not copyrighted. They are in the public domain and may be republished, reprinted and otherwise used freely by anyone without the need to obtain permission from FDA. Credit to the U.S. Food and Drug Administration as the source is appreciated but not required. | |
Record name | Dihydrouracil | |
Source | Human Metabolome Database (HMDB) | |
URL | http://www.hmdb.ca/metabolites/HMDB0000076 | |
Description | The Human Metabolome Database (HMDB) is a freely available electronic database containing detailed information about small molecule metabolites found in the human body. | |
Explanation | HMDB is offered to the public as a freely available resource. Use and re-distribution of the data, in whole or in part, for commercial purposes requires explicit permission of the authors and explicit acknowledgment of the source material (HMDB) and the original publication (see the HMDB citing page). We ask that users who download significant portions of the database cite the HMDB paper in any resulting publications. | |
Melting Point |
279 - 281 °C | |
Record name | Dihydrouracil | |
Source | Human Metabolome Database (HMDB) | |
URL | http://www.hmdb.ca/metabolites/HMDB0000076 | |
Description | The Human Metabolome Database (HMDB) is a freely available electronic database containing detailed information about small molecule metabolites found in the human body. | |
Explanation | HMDB is offered to the public as a freely available resource. Use and re-distribution of the data, in whole or in part, for commercial purposes requires explicit permission of the authors and explicit acknowledgment of the source material (HMDB) and the original publication (see the HMDB citing page). We ask that users who download significant portions of the database cite the HMDB paper in any resulting publications. | |
Retrosynthesis Analysis
AI-Powered Synthesis Planning: Our tool employs the Template_relevance Pistachio, Template_relevance Bkms_metabolic, Template_relevance Pistachio_ringbreaker, Template_relevance Reaxys, Template_relevance Reaxys_biocatalysis model, leveraging a vast database of chemical reactions to predict feasible synthetic routes.
One-Step Synthesis Focus: Specifically designed for one-step synthesis, it provides concise and direct routes for your target compounds, streamlining the synthesis process.
Accurate Predictions: Utilizing the extensive PISTACHIO, BKMS_METABOLIC, PISTACHIO_RINGBREAKER, REAXYS, REAXYS_BIOCATALYSIS database, our tool offers high-accuracy predictions, reflecting the latest in chemical research and data.
Strategy Settings
Precursor scoring | Relevance Heuristic |
---|---|
Min. plausibility | 0.01 |
Model | Template_relevance |
Template Set | Pistachio/Bkms_metabolic/Pistachio_ringbreaker/Reaxys/Reaxys_biocatalysis |
Top-N result to add to graph | 6 |
Feasible Synthetic Routes
Q1: What is the role of Dihydrouracil in pyrimidine catabolism?
A1: Dihydrouracil is a crucial intermediate in the degradation pathway of uracil and thymine. [] It is formed by the NADPH-dependent reduction of uracil, catalyzed by the enzyme dihydropyrimidine dehydrogenase (DPD). [] This step is the rate-limiting step in the pyrimidine degradation pathway. [] Further enzymatic reactions convert dihydrouracil into β-alanine, ammonia, and CO2. []
Q2: How does Dihydrouracil relate to 5-Fluorouracil (5-FU) chemotherapy?
A2: DPD, the enzyme responsible for Dihydrouracil formation, also metabolizes the chemotherapeutic drug 5-FU. [] Individuals with DPD deficiency are at increased risk of severe toxicity from 5-FU treatment. [] Monitoring the ratio of dihydrouracil to uracil (DHU/U) can provide insights into an individual's DPD activity and guide 5-FU dosage adjustments. []
Q3: Can Dihydrouracil levels predict adverse reactions to pyrimidine chemotherapy?
A3: Measuring urinary pyrimidine levels, including dihydrouracil, could potentially identify individuals with abnormal pyrimidine metabolism, like dihydropyrimidinase deficiency. [] This could help predict potential adverse reactions to pyrimidine-based chemotherapy. []
Q4: How does Dihydrouracil relate to dihydropyrimidinase deficiency?
A4: Dihydropyrimidinase deficiency, a rare metabolic disorder, leads to the accumulation of dihydrouracil and dihydrothymine in urine (dihydropyrimidinuria). [, ] This condition can be diagnosed by analyzing urinary pyrimidine profiles, revealing elevated dihydrouracil levels. []
Q5: What is the molecular formula and weight of Dihydrouracil?
A5: Dihydrouracil has the molecular formula C4H6N2O2 and a molecular weight of 114.10 g/mol.
Q6: How can spectroscopic techniques be used to characterize Dihydrouracil?
A6: Nuclear Magnetic Resonance (NMR) spectroscopy, particularly 1H and 13C NMR, provides detailed structural information about Dihydrouracil. [, ] Electron Spin Resonance (ESR) techniques have been used to study radiation-induced radicals in Dihydrouracil crystals. []
Q7: How stable is Dihydrouracil under different conditions?
A7: Dihydrouracil exhibits varying stability depending on the conditions. It is relatively stable in a dry state but undergoes degradation in aqueous solutions, particularly at elevated temperatures. [] The rate of ring opening increases with temperature, with a half-life of 9.1 hours at 100°C and pH 7. [] This instability at high temperatures may explain the absence of dihydrouridine, the nucleoside form of dihydrouracil, from the tRNA of hyperthermophiles. []
Q8: What is the effect of allylic strain on the ring opening of Dihydrouracil derivatives?
A8: Studies on the alkaline hydrolysis of dihydrouracil derivatives have shown that introducing a 1-nitrogen substituent significantly decreases the ring-opening rate. [] This effect is attributed to allylic strain, similar to the gem-dimethyl effect on ring closure. []
Q9: How does pH influence the radiolysis products of Dihydrouracil in the presence of oxygen?
A9: The products formed during the radiolysis of dihydrouracil in aerated solutions depend on both pH and dose rate. [] At acidic pH and low dose rates, barbituric acid is a major product, while uracil becomes predominant at alkaline pH and higher dose rates. [] This difference arises from the base-catalyzed elimination of superoxide radicals (O2•−) from the 6-peroxyl radical of dihydrouracil, leading to uracil formation. []
Q10: What is the role of isopyrimidines in the radiolysis of Dihydrouracil?
A10: During the radiolysis of dihydrouracil, the 6-peroxyl radical can undergo an elimination reaction to form uracil via an unstable isomeric form of the pyrimidine, known as isouracil. [] This reaction, which releases a superoxide radical, is base-catalyzed, explaining the pH dependence of the product distribution in irradiated dihydrouracil solutions. []
Q11: What analytical methods are available for Dihydrouracil quantification?
A11: Several analytical techniques have been developed for quantifying dihydrouracil in biological samples. These include high-performance liquid chromatography (HPLC) with ultraviolet (UV) detection, [, , ] HPLC coupled with tandem mass spectrometry (HPLC-MS/MS), [, , ] and gas chromatography-mass spectrometry (GC-MS). [] The choice of method depends on factors like sensitivity, specificity, and available instrumentation.
Q12: How is computational chemistry used to study Dihydrouracil and related compounds?
A12: Computational methods like molecular docking and molecular dynamics simulations help investigate the interactions of Dihydrouracil and its derivatives with enzymes like dihydropyrimidine dehydrogenase. [] These studies provide insights into binding affinities, molecular mechanisms, and potential for developing enzyme inhibitors. []
Q13: What are the Structure-Activity Relationships (SAR) of Dihydrouracil analogs as enzyme inhibitors?
A13: Studies have explored the SAR of dihydrouracil analogs as potential inhibitors of enzymes involved in pyrimidine metabolism, such as dihydropyrimidine dehydrogenase, dihydroorotase, and dihydroorotate dehydrogenase. [] By modifying the dihydrouracil structure, researchers aim to enhance binding affinity, selectivity, and inhibitory potency for these enzymes. []
Q14: What are the potential applications of Dihydrouracil research?
A14: Research on Dihydrouracil and its metabolic pathway contributes to a better understanding of pyrimidine metabolism and related disorders. [] This knowledge aids in developing diagnostic tools and therapeutic strategies for managing diseases like cancer and metabolic disorders. []
Q15: How can the knowledge of Dihydrouracil metabolism improve cancer treatment?
A15: Understanding Dihydrouracil metabolism, particularly its link to DPD activity, allows for personalized 5-FU chemotherapy. [, ] By determining an individual's DHU/U ratio, clinicians can adjust 5-FU dosage, minimizing the risk of severe toxicity and improving treatment outcomes. [, ]
Q16: What is the significance of Dihydrouracil in the context of DNA damage and repair?
A16: Dihydrouracil can be formed in DNA as a result of oxidative damage to cytosine. [] This lesion is primarily repaired by the base excision repair (BER) pathway, involving enzymes like endonuclease III. [] Understanding the repair mechanisms of dihydrouracil in DNA contributes to our knowledge of genome stability and mutagenesis. []
体外研究产品的免责声明和信息
请注意,BenchChem 上展示的所有文章和产品信息仅供信息参考。 BenchChem 上可购买的产品专为体外研究设计,这些研究在生物体外进行。体外研究,源自拉丁语 "in glass",涉及在受控实验室环境中使用细胞或组织进行的实验。重要的是要注意,这些产品没有被归类为药物或药品,他们没有得到 FDA 的批准,用于预防、治疗或治愈任何医疗状况、疾病或疾病。我们必须强调,将这些产品以任何形式引入人类或动物的身体都是法律严格禁止的。遵守这些指南对确保研究和实验的法律和道德标准的符合性至关重要。