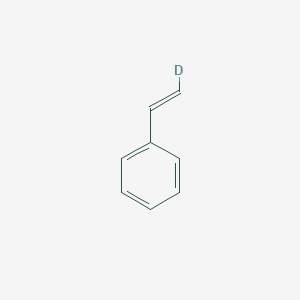
2-氘乙烯基苯
概述
描述
2-Deuterioethenylbenzene is a deuterated derivative of ethenylbenzene, where one of the hydrogen atoms in the ethenyl group is replaced by deuterium. This compound is of interest due to its unique isotopic properties, which can influence its chemical behavior and physical properties. Deuterium, being a heavier isotope of hydrogen, can affect reaction kinetics and mechanisms, making 2-Deuterioethenylbenzene a valuable compound in various scientific studies.
科学研究应用
2-Deuterioethenylbenzene has a wide range of applications in scientific research:
Chemistry: It is used as a tracer in reaction mechanism studies due to its isotopic labeling.
Biology: The compound can be used in metabolic studies to understand the pathways and mechanisms of biological processes involving ethenylbenzene derivatives.
准备方法
Synthetic Routes and Reaction Conditions: The synthesis of 2-Deuterioethenylbenzene typically involves the deuteration of ethenylbenzene. One common method is the hydrogen-deuterium exchange reaction, where ethenylbenzene is treated with deuterium gas in the presence of a catalyst. This process can be carried out under high temperatures and pressures to facilitate the exchange of hydrogen atoms with deuterium .
Industrial Production Methods: Industrial production of 2-Deuterioethenylbenzene may involve large-scale deuteration processes using deuterium gas and suitable catalysts. The reaction conditions are optimized to achieve high yields and purity of the deuterated product. The use of perdeuterated solvents and reagents can further enhance the efficiency of the deuteration process .
化学反应分析
Types of Reactions: 2-Deuterioethenylbenzene can undergo various chemical reactions, including:
Oxidation: The benzylic position in 2-Deuterioethenylbenzene can be oxidized to form benzoic acid derivatives.
Reduction: Reduction reactions can convert 2-Deuterioethenylbenzene to deuterated ethylbenzene using reducing agents like lithium aluminum hydride (LiAlH₄).
Common Reagents and Conditions:
Oxidation: Potassium permanganate (KMnO₄), acidic conditions.
Reduction: Lithium aluminum hydride (LiAlH₄), anhydrous conditions.
Substitution: Electrophiles such as halogens, catalysts like aluminum chloride (AlCl₃).
Major Products:
Oxidation: Benzoic acid derivatives.
Reduction: Deuterated ethylbenzene.
Substitution: Various substituted ethenylbenzene derivatives.
作用机制
The mechanism of action of 2-Deuterioethenylbenzene involves its interaction with various molecular targets and pathways. The presence of deuterium can alter the bond strengths and reaction kinetics, leading to different reaction pathways compared to non-deuterated ethenylbenzene. This isotopic effect is particularly useful in studying reaction mechanisms and understanding the role of hydrogen atoms in chemical and biological processes .
相似化合物的比较
Ethenylbenzene (Styrene): The non-deuterated counterpart of 2-Deuterioethenylbenzene.
Deuterated Benzene: Another deuterated aromatic compound used in similar studies.
Deuterated Ethylbenzene: A deuterated derivative of ethylbenzene.
Uniqueness: 2-Deuterioethenylbenzene is unique due to its specific isotopic substitution, which provides distinct advantages in isotopic labeling studies. The presence of deuterium can significantly influence the reaction kinetics and mechanisms, making it a valuable tool in both fundamental and applied research .
属性
IUPAC Name |
2-deuterioethenylbenzene | |
---|---|---|
Source | PubChem | |
URL | https://pubchem.ncbi.nlm.nih.gov | |
Description | Data deposited in or computed by PubChem | |
InChI |
InChI=1S/C8H8/c1-2-8-6-4-3-5-7-8/h2-7H,1H2/i1D | |
Source | PubChem | |
URL | https://pubchem.ncbi.nlm.nih.gov | |
Description | Data deposited in or computed by PubChem | |
InChI Key |
PPBRXRYQALVLMV-MICDWDOJSA-N | |
Source | PubChem | |
URL | https://pubchem.ncbi.nlm.nih.gov | |
Description | Data deposited in or computed by PubChem | |
Canonical SMILES |
C=CC1=CC=CC=C1 | |
Source | PubChem | |
URL | https://pubchem.ncbi.nlm.nih.gov | |
Description | Data deposited in or computed by PubChem | |
Isomeric SMILES |
[2H]C=CC1=CC=CC=C1 | |
Source | PubChem | |
URL | https://pubchem.ncbi.nlm.nih.gov | |
Description | Data deposited in or computed by PubChem | |
Molecular Formula |
C8H8 | |
Source | PubChem | |
URL | https://pubchem.ncbi.nlm.nih.gov | |
Description | Data deposited in or computed by PubChem | |
Molecular Weight |
105.15 g/mol | |
Source | PubChem | |
URL | https://pubchem.ncbi.nlm.nih.gov | |
Description | Data deposited in or computed by PubChem | |
CAS No. |
21370-59-2, 6911-81-5 | |
Record name | cis-Styrene-(β )-d | |
Source | European Chemicals Agency (ECHA) | |
URL | https://echa.europa.eu/information-on-chemicals | |
Description | The European Chemicals Agency (ECHA) is an agency of the European Union which is the driving force among regulatory authorities in implementing the EU's groundbreaking chemicals legislation for the benefit of human health and the environment as well as for innovation and competitiveness. | |
Explanation | Use of the information, documents and data from the ECHA website is subject to the terms and conditions of this Legal Notice, and subject to other binding limitations provided for under applicable law, the information, documents and data made available on the ECHA website may be reproduced, distributed and/or used, totally or in part, for non-commercial purposes provided that ECHA is acknowledged as the source: "Source: European Chemicals Agency, http://echa.europa.eu/". Such acknowledgement must be included in each copy of the material. ECHA permits and encourages organisations and individuals to create links to the ECHA website under the following cumulative conditions: Links can only be made to webpages that provide a link to the Legal Notice page. | |
Record name | trans-Styrene-(β )-d | |
Source | European Chemicals Agency (ECHA) | |
URL | https://echa.europa.eu/information-on-chemicals | |
Description | The European Chemicals Agency (ECHA) is an agency of the European Union which is the driving force among regulatory authorities in implementing the EU's groundbreaking chemicals legislation for the benefit of human health and the environment as well as for innovation and competitiveness. | |
Explanation | Use of the information, documents and data from the ECHA website is subject to the terms and conditions of this Legal Notice, and subject to other binding limitations provided for under applicable law, the information, documents and data made available on the ECHA website may be reproduced, distributed and/or used, totally or in part, for non-commercial purposes provided that ECHA is acknowledged as the source: "Source: European Chemicals Agency, http://echa.europa.eu/". Such acknowledgement must be included in each copy of the material. ECHA permits and encourages organisations and individuals to create links to the ECHA website under the following cumulative conditions: Links can only be made to webpages that provide a link to the Legal Notice page. | |
Synthesis routes and methods I
Procedure details
Synthesis routes and methods II
Procedure details
Synthesis routes and methods III
Procedure details
Synthesis routes and methods IV
Procedure details
Synthesis routes and methods V
Procedure details
体外研究产品的免责声明和信息
请注意,BenchChem 上展示的所有文章和产品信息仅供信息参考。 BenchChem 上可购买的产品专为体外研究设计,这些研究在生物体外进行。体外研究,源自拉丁语 "in glass",涉及在受控实验室环境中使用细胞或组织进行的实验。重要的是要注意,这些产品没有被归类为药物或药品,他们没有得到 FDA 的批准,用于预防、治疗或治愈任何医疗状况、疾病或疾病。我们必须强调,将这些产品以任何形式引入人类或动物的身体都是法律严格禁止的。遵守这些指南对确保研究和实验的法律和道德标准的符合性至关重要。