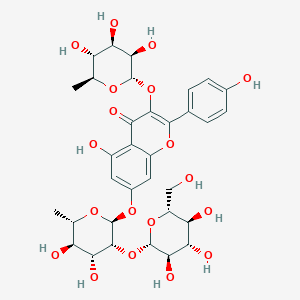
Grosvenorine
作用机制
罗汉果甜苷通过多种机制发挥作用:
抗菌活性: 它破坏细菌细胞壁并抑制必要的细菌酶,导致细菌细胞死亡.
抗氧化活性: 它清除自由基并上调抗氧化酶,保护细胞免受氧化应激.
抗炎活性: 它抑制促炎细胞因子并调节炎症途径,减少炎症.
生化分析
Biochemical Properties
Grosvenorine plays a crucial role in biochemical reactions, particularly due to its interactions with various enzymes, proteins, and other biomolecules. It has been observed to interact with enzymes involved in antioxidant activities, such as superoxide dismutase (SOD) and catalase. These interactions help in neutralizing free radicals and reducing oxidative stress within cells . Additionally, this compound has been found to interact with proteins involved in inflammatory pathways, thereby exhibiting anti-inflammatory properties.
Cellular Effects
This compound exerts significant effects on various types of cells and cellular processes. It has been shown to influence cell function by modulating cell signaling pathways, gene expression, and cellular metabolism. For instance, this compound can activate the nuclear factor erythroid 2-related factor 2 (Nrf2) pathway, leading to the upregulation of antioxidant response elements and enhancing the cell’s defense mechanisms against oxidative stress . Furthermore, this compound has been reported to inhibit the expression of pro-inflammatory cytokines, thereby reducing inflammation at the cellular level.
Molecular Mechanism
The molecular mechanism of this compound involves its binding interactions with biomolecules, enzyme inhibition or activation, and changes in gene expression. This compound binds to specific receptors on the cell surface, initiating a cascade of intracellular signaling events. It can inhibit the activity of enzymes such as cyclooxygenase (COX) and lipoxygenase (LOX), which are involved in the production of inflammatory mediators . Additionally, this compound can modulate the expression of genes related to antioxidant defense and inflammation, thereby exerting its protective effects at the molecular level.
Temporal Effects in Laboratory Settings
In laboratory settings, the effects of this compound have been observed to change over time. The compound exhibits stability under controlled conditions, but its degradation can occur under extreme pH or temperature conditions. Long-term studies have shown that this compound can maintain its antioxidant and anti-inflammatory effects over extended periods, both in vitro and in vivo . The extent of these effects may vary depending on the experimental conditions and the duration of exposure.
Dosage Effects in Animal Models
The effects of this compound vary with different dosages in animal models. At lower doses, this compound has been found to exhibit beneficial effects such as enhanced antioxidant activity and reduced inflammation. At higher doses, there may be threshold effects, leading to potential toxicity or adverse effects . It is crucial to determine the optimal dosage range to maximize the therapeutic benefits of this compound while minimizing any potential risks.
Metabolic Pathways
This compound is involved in various metabolic pathways, interacting with enzymes and cofactors that regulate metabolic flux and metabolite levels. It has been shown to enhance the activity of enzymes involved in the detoxification of reactive oxygen species (ROS), thereby reducing oxidative damage . Additionally, this compound can influence the levels of metabolites involved in inflammatory pathways, further contributing to its anti-inflammatory effects.
Transport and Distribution
Within cells and tissues, this compound is transported and distributed through specific transporters and binding proteins. These interactions facilitate its localization and accumulation in target tissues, where it can exert its biological effects . The transport and distribution of this compound are crucial for its bioavailability and therapeutic efficacy.
Subcellular Localization
This compound exhibits specific subcellular localization, which can impact its activity and function. It has been observed to localize in the cytoplasm and nucleus, where it can interact with various cellular components . Post-translational modifications and targeting signals play a role in directing this compound to specific compartments or organelles, thereby influencing its biological activity.
准备方法
合成路线和反应条件: 罗汉果甜苷的合成涉及从罗汉果果实中提取该化合物。该过程通常包括:
提取: 使用乙醇或甲醇等溶剂从干燥的果实中提取黄酮类化合物。
纯化: 采用柱层析等技术从粗提物中纯化罗汉果甜苷。
工业生产方法: 罗汉果甜苷的工业生产侧重于优化提取和纯化过程,以确保高产率和纯度。这通常涉及:
大规模提取: 利用工业溶剂和先进的提取技术。
纯化: 采用高效液相色谱 (HPLC) 进行大规模纯化。
化学反应分析
反应类型: 罗汉果甜苷经历各种化学反应,包括:
氧化: 罗汉果甜苷可以被氧化形成不同的氧化产物,这些产物可能具有不同的生物活性。
还原: 还原反应可以改变黄酮类化合物的结构,可能改变其生物学特性。
取代: 取代反应,特别是涉及羟基的反应,可以导致形成具有不同活性的衍生物。
常用试剂和条件:
氧化: 常见的氧化剂包括过氧化氢和高锰酸钾。
还原: 硼氢化钠等还原剂经常使用。
取代: 乙酸酐和硫酸等试剂用于乙酰化反应。
主要产物: 这些反应形成的主要产物包括罗汉果甜苷的各种氧化、还原和取代衍生物,每个衍生物都可能具有独特的生物活性。
科学研究应用
罗汉果甜苷在科学研究中具有广泛的应用:
化学: 用作模型化合物来研究黄酮类化合物的化学性质和反应。
生物学: 研究它在细胞过程中的作用及其对各种生物途径的影响。
医学: 探索其潜在的治疗效果,包括抗菌、抗氧化、抗炎和抗癌活性
工业: 由于其有益特性,用于开发天然保健产品和补充剂。
相似化合物的比较
罗汉果甜苷在黄酮类化合物中是独特的,因为它具有独特的结构和生物活性。类似的化合物包括:
槲皮素: 另一种具有强抗氧化和抗炎特性的黄酮类化合物。
山奈酚: 以其抗癌和心血管保护作用而闻名。
木犀草素: 具有抗炎和神经保护活性。
属性
IUPAC Name |
7-[(2S,3R,4R,5R,6S)-4,5-dihydroxy-6-methyl-3-[(2S,3R,4S,5S,6R)-3,4,5-trihydroxy-6-(hydroxymethyl)oxan-2-yl]oxyoxan-2-yl]oxy-5-hydroxy-2-(4-hydroxyphenyl)-3-[(2S,3R,4R,5R,6S)-3,4,5-trihydroxy-6-methyloxan-2-yl]oxychromen-4-one | |
---|---|---|
Source | PubChem | |
URL | https://pubchem.ncbi.nlm.nih.gov | |
Description | Data deposited in or computed by PubChem | |
InChI |
InChI=1S/C33H40O19/c1-10-19(37)23(41)26(44)31(46-10)51-29-22(40)18-15(36)7-14(8-16(18)49-28(29)12-3-5-13(35)6-4-12)48-33-30(25(43)20(38)11(2)47-33)52-32-27(45)24(42)21(39)17(9-34)50-32/h3-8,10-11,17,19-21,23-27,30-39,41-45H,9H2,1-2H3/t10-,11-,17+,19-,20-,21+,23+,24-,25+,26+,27+,30+,31-,32-,33-/m0/s1 | |
Source | PubChem | |
URL | https://pubchem.ncbi.nlm.nih.gov | |
Description | Data deposited in or computed by PubChem | |
InChI Key |
FFGGWIVHOGEVSP-NWQOLJAQSA-N | |
Source | PubChem | |
URL | https://pubchem.ncbi.nlm.nih.gov | |
Description | Data deposited in or computed by PubChem | |
Canonical SMILES |
CC1C(C(C(C(O1)OC2=C(OC3=CC(=CC(=C3C2=O)O)OC4C(C(C(C(O4)C)O)O)OC5C(C(C(C(O5)CO)O)O)O)C6=CC=C(C=C6)O)O)O)O | |
Source | PubChem | |
URL | https://pubchem.ncbi.nlm.nih.gov | |
Description | Data deposited in or computed by PubChem | |
Isomeric SMILES |
C[C@H]1[C@@H]([C@H]([C@H]([C@@H](O1)OC2=C(OC3=CC(=CC(=C3C2=O)O)O[C@H]4[C@@H]([C@@H]([C@H]([C@@H](O4)C)O)O)O[C@H]5[C@@H]([C@H]([C@@H]([C@H](O5)CO)O)O)O)C6=CC=C(C=C6)O)O)O)O | |
Source | PubChem | |
URL | https://pubchem.ncbi.nlm.nih.gov | |
Description | Data deposited in or computed by PubChem | |
Molecular Formula |
C33H40O19 | |
Source | PubChem | |
URL | https://pubchem.ncbi.nlm.nih.gov | |
Description | Data deposited in or computed by PubChem | |
Molecular Weight |
740.7 g/mol | |
Source | PubChem | |
URL | https://pubchem.ncbi.nlm.nih.gov | |
Description | Data deposited in or computed by PubChem | |
Retrosynthesis Analysis
AI-Powered Synthesis Planning: Our tool employs the Template_relevance Pistachio, Template_relevance Bkms_metabolic, Template_relevance Pistachio_ringbreaker, Template_relevance Reaxys, Template_relevance Reaxys_biocatalysis model, leveraging a vast database of chemical reactions to predict feasible synthetic routes.
One-Step Synthesis Focus: Specifically designed for one-step synthesis, it provides concise and direct routes for your target compounds, streamlining the synthesis process.
Accurate Predictions: Utilizing the extensive PISTACHIO, BKMS_METABOLIC, PISTACHIO_RINGBREAKER, REAXYS, REAXYS_BIOCATALYSIS database, our tool offers high-accuracy predictions, reflecting the latest in chemical research and data.
Strategy Settings
Precursor scoring | Relevance Heuristic |
---|---|
Min. plausibility | 0.01 |
Model | Template_relevance |
Template Set | Pistachio/Bkms_metabolic/Pistachio_ringbreaker/Reaxys/Reaxys_biocatalysis |
Top-N result to add to graph | 6 |
Feasible Synthetic Routes
体外研究产品的免责声明和信息
请注意,BenchChem 上展示的所有文章和产品信息仅供信息参考。 BenchChem 上可购买的产品专为体外研究设计,这些研究在生物体外进行。体外研究,源自拉丁语 "in glass",涉及在受控实验室环境中使用细胞或组织进行的实验。重要的是要注意,这些产品没有被归类为药物或药品,他们没有得到 FDA 的批准,用于预防、治疗或治愈任何医疗状况、疾病或疾病。我们必须强调,将这些产品以任何形式引入人类或动物的身体都是法律严格禁止的。遵守这些指南对确保研究和实验的法律和道德标准的符合性至关重要。