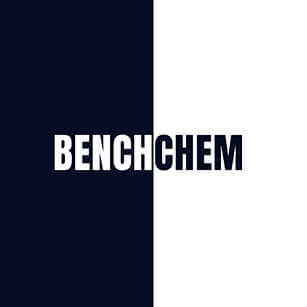
羧肽酶 A
- 点击 快速询问 获取最新报价。
- 提供有竞争力价格的高质量产品,您可以更专注于研究。
描述
Carboxypeptidase A is a zinc-dependent metalloenzyme primarily found in the pancreas of mammals. It plays a crucial role in the digestion of proteins by cleaving the C-terminal amino acid residues from polypeptide chains. This enzyme is highly specific for substrates with aromatic or aliphatic side chains, such as phenylalanine, tryptophan, and leucine .
科学研究应用
Carboxypeptidase A has a wide range of applications in scientific research:
作用机制
Target of Action
Carboxypeptidase A (CPA) is a pancreatic exopeptidase that hydrolyzes peptide bonds of C-terminal residues with aromatic or aliphatic side-chains . It is also referred to as CPA1 . The enzyme’s primary targets are proteins and peptides, specifically at the C-terminal end . It cleaves only L-amino acids with free carboxyl groups adjacent to the peptide or ester bond and is specific for amino acids that have aromatic or hydrophobic side chains, such as phenylalanine, tryptophan, or leucine .
Mode of Action
CPA employs a zinc ion within the protein for hydrolysis of the peptide bond at the C-terminal end of an amino acid residue . Loss of the zinc leads to loss of activity, which can be replaced easily by zinc, and also by some other divalent metals (cobalt, nickel) . The zinc-bound hydroxide interacts with the amide with the electrostatic stabilization of the transition state provided by the Zn 2+ ion and the neighbouring arginine .
Biochemical Pathways
CPA facilitates the breakdown of proteins during metabolism . It is involved in the digestion of proteins by mast cells . CPA is crucial to many processes in the human body, including digestion, post-translational modification of proteins, blood clotting, and reproduction .
Pharmacokinetics
It is known that cpa is produced in the pancreas . The enzyme’s activity can be influenced by the presence of zinc and other divalent metals .
Result of Action
The result of CPA’s action is the hydrolysis of peptide bonds at the C-terminal end of proteins and peptides . This enzymatic action results in the breakdown of proteins during metabolism , aiding in digestion and other biological processes .
Action Environment
CPA is present in the extracellular matrix where it is enzymatically active . The enzyme’s activity can be influenced by environmental factors such as the presence of zinc and other divalent metals .
生化分析
Biochemical Properties
Carboxypeptidase A functions as an exopeptidase, cleaving amino acids from the C-terminal end of polypeptides. It interacts with several biomolecules, including zinc ions, which are essential for its catalytic activity. The enzyme’s active site contains a zinc ion coordinated by amino acid residues such as histidine and glutamate, which facilitate the hydrolysis of peptide bonds . Carboxypeptidase A also interacts with substrates like glycyl-L-tyrosine and inhibitors such as β-(p-iodophenyl)propionate .
Cellular Effects
Carboxypeptidase A influences various cellular processes by modulating protein and peptide levels. It plays a role in cell signaling pathways, gene expression, and cellular metabolism. For instance, carboxypeptidase A is involved in the maturation of neuroendocrine peptides, which are critical for cell signaling . Additionally, it affects the degradation of extracellular matrix proteins, impacting cellular migration and tissue remodeling .
Molecular Mechanism
The molecular mechanism of carboxypeptidase A involves the hydrolysis of peptide bonds at the C-terminal end of substrates. The enzyme’s active site contains a zinc ion that coordinates with water molecules, facilitating nucleophilic attack on the peptide bond . This process is stabilized by interactions with amino acid residues such as arginine and tyrosine, which enhance substrate binding and catalysis . Carboxypeptidase A can also be inhibited by molecules that bind to its active site, preventing substrate access .
Temporal Effects in Laboratory Settings
In laboratory settings, the activity of carboxypeptidase A can change over time due to factors such as enzyme stability and degradation. Studies have shown that crystallization can reduce the enzyme’s activity, but it remains stable for extended periods under specific conditions . Long-term effects on cellular function have been observed, including alterations in protein degradation and peptide maturation .
Dosage Effects in Animal Models
The effects of carboxypeptidase A vary with different dosages in animal models. At optimal doses, the enzyme facilitates normal physiological processes such as digestion and protein metabolism. At high doses, carboxypeptidase A can cause adverse effects, including tissue damage and inflammation . Threshold effects have been observed, indicating that precise dosage control is essential for therapeutic applications .
Metabolic Pathways
Carboxypeptidase A is involved in several metabolic pathways, including protein catabolism and peptide maturation. It interacts with enzymes such as trypsin and chymotrypsin, which activate its zymogen form in the pancreas . The enzyme also participates in the renin-angiotensin-aldosterone system, influencing blood pressure regulation .
Transport and Distribution
Within cells and tissues, carboxypeptidase A is transported and distributed through secretory pathways. It is synthesized in the pancreas and secreted into the digestive tract, where it acts on dietary proteins . The enzyme’s distribution is regulated by transporters and binding proteins that ensure its localization to specific cellular compartments .
Subcellular Localization
Carboxypeptidase A is localized in the lumen of secretory organelles such as the endoplasmic reticulum and Golgi apparatus. It is directed to these compartments by targeting signals and post-translational modifications . The enzyme’s activity is influenced by its subcellular localization, with optimal function occurring in the acidic environment of secretory granules .
准备方法
Synthetic Routes and Reaction Conditions: Carboxypeptidase A is typically isolated from bovine pancreas through a series of purification steps, including ammonium sulfate precipitation, ion-exchange chromatography, and gel filtration . The enzyme is then crystallized to achieve a high degree of purity.
Industrial Production Methods: In industrial settings, carboxypeptidase A is produced using recombinant DNA technology. The gene encoding the enzyme is inserted into a suitable expression system, such as Escherichia coli or yeast, which then produces the enzyme in large quantities. The recombinant enzyme is purified using similar techniques as those used for the natural enzyme .
化学反应分析
Types of Reactions: Carboxypeptidase A primarily catalyzes hydrolysis reactions, specifically the cleavage of peptide bonds at the C-terminal end of polypeptides . It can also hydrolyze esters under certain experimental conditions .
Common Reagents and Conditions: The enzyme requires a zinc ion (Zn²⁺) at its active site for catalytic activity. The optimal pH for its activity is around 7.5, and it functions best at physiological temperatures (37°C) .
Major Products: The primary products of carboxypeptidase A-catalyzed reactions are free amino acids and shorter peptide fragments .
相似化合物的比较
Carboxypeptidase A is part of a larger family of carboxypeptidases, including carboxypeptidase B, carboxypeptidase N, and carboxypeptidase Y. Each of these enzymes has unique substrate specificities and biological functions:
Carboxypeptidase B: Prefers substrates with basic amino acids like arginine and lysine.
Carboxypeptidase N: Involved in the regulation of peptide hormones and the complement system.
Carboxypeptidase Y: Found in yeast and involved in protein maturation.
Carboxypeptidase A is unique due to its preference for aromatic and aliphatic amino acids and its role in digestive processes .
属性
CAS 编号 |
11075-17-5 |
---|---|
分子式 |
C12H25NO4 |
分子量 |
247.33 g/mol |
IUPAC 名称 |
2-hexyl-6-(hydroxymethyl)piperidine-3,4,5-triol |
InChI |
InChI=1S/C12H25NO4/c1-2-3-4-5-6-8-10(15)12(17)11(16)9(7-14)13-8/h8-17H,2-7H2,1H3 |
InChI 键 |
CNCWFVJLRHKDJX-UHFFFAOYSA-N |
规范 SMILES |
CCCCCCC1C(C(C(C(N1)CO)O)O)O |
产品来源 |
United States |
Q1: How does the conformation of Carboxypeptidase A (CPA) differ in solution and crystalline states, and what implications does this have on its activity?
A1: [, , ] Studies utilizing spectroscopic probes like arsanilazotyrosine-248 have revealed distinct conformational differences between CPA in solution and its crystalline form. Specifically, the orientation of key residues like Tyr-248 relative to the active-site zinc ion varies between these states. This difference in conformation significantly impacts the enzyme's catalytic efficiency (kcat). Crystallization consistently leads to a substantial decrease in kcat, ranging from 20- to 1000-fold for various substrates, compared to CPA in solution. This underscores the critical role of conformational dynamics in CPA's function. For a deeper understanding, refer to the research paper focusing on the kinetic properties of crystalline enzymes [].
Q2: Can the presence of substrates or inhibitors influence the conformation of CPA?
A2: [] Yes, the interaction of CPA with substrates, inhibitors, or even changes in pH can induce conformational shifts in the enzyme. These shifts are particularly noticeable in the orientation of the arsanilazotyrosine-248 side chain, highlighting the dynamic nature of the active site in response to its chemical environment.
Q3: What is the significance of the arsanilazotyrosine-248-zinc complex in studying CPA?
A3: [] This complex serves as a sensitive probe for monitoring changes in CPA's environment. Alterations in pH or the physical state of the enzyme (solution vs. crystal) trigger noticeable spectral changes in the complex, providing insights into the dynamic behavior of CPA.
Q4: Does human pancreatic tissue express multiple forms of Carboxypeptidase A?
A4: [] Yes, research has identified two distinct isoenzymes of Carboxypeptidase A in human pancreatic tissue. These isoenzymes, while similar in structure, exhibit only 61% homology in their first 31 amino-terminal amino acids, showcasing a degree of diversity in CPA forms within humans.
Q5: Are there significant structural similarities between human Carboxypeptidase A2 and its counterparts in other species?
A5: [] Yes, the amino acid sequence of human Carboxypeptidase A2 shares a high degree of similarity with other species. It has 89% identity with rat procarboxypeptidase A2 and 64% identity with human procarboxypeptidase A1. This high degree of conservation suggests a common evolutionary origin and a conserved function across species.
Q6: What is the role of the pro-segment in the structure of human procarboxypeptidase A2?
A6: [] The pro-segment of human procarboxypeptidase A2, consisting of 96 residues, plays a crucial role in regulating the enzyme's activity. This segment acts as an inhibitor by blocking the substrate binding sites (S2-S4) in the enzyme's active site. The interaction between the globular part of the pro-segment and the enzyme moiety effectively shields the active site, preventing premature activation.
Q7: How does the structure of the pro-segment in human procarboxypeptidase A2 compare to that of other procarboxypeptidases, and what are the implications of these differences?
A7: [] The structure of the pro-segment in human procarboxypeptidase A2, while sharing similarities with other procarboxypeptidases, exhibits unique features that contribute to the distinct activation behavior observed in procarboxypeptidases A1, B, and A2. These structural variations within the pro-segment are likely responsible for the differences in their activation processes.
Q8: How does the stability of porcine procarboxypeptidase A compare to that of its isolated activation segment?
A8: [] Differential scanning calorimetry studies revealed distinct thermal stability profiles for porcine procarboxypeptidase A and its isolated activation segment. While the zymogen and the active enzyme exhibit irreversible thermal denaturation, the isolated activation segment demonstrates high thermostability and reversible thermal unfolding. This difference highlights the independent folding and stability characteristics of the activation segment.
Q9: Does cobalt procarboxypeptidase A exhibit catalytic activity?
A9: [] Yes, cobalt procarboxypeptidase A demonstrates substantial peptidase activity towards a range of halogen-substituted acylamino acids. Surprisingly, its activity equals or even surpasses that of the native zinc enzyme. This discovery challenges the traditional view of zymogen activation, suggesting that the catalytic machinery is largely pre-formed in the zymogen and requires minimal conformational changes for activation.
Q10: How do halides influence the dipeptidyl and tripeptidyl carboxypeptidase activities of kininase II?
A10: [] Halides exhibit varied effects on the catalytic activities of kininase II. While fluoride ions (F-) act as activators for the dipeptidyl carboxypeptidase activity, particularly with bradykinin as the substrate, other halides (Cl-, Br-, I-) have minimal impact on this activity. Interestingly, all halides enhance the tripeptidyl carboxypeptidase activity of kininase II, showcasing the complex regulatory role of halides on this enzyme.
Q11: What is the role of tubulin carboxypeptidase, and how is its activity regulated within cells?
A11: [] Tubulin carboxypeptidase plays a critical role in regulating the tyrosination state of microtubules by removing the C-terminal tyrosine from alpha-tubulin. Interestingly, this enzyme is not uniformly distributed within cells. Instead, it associates specifically with cold-labile microtubules, and this association is influenced by the cell density, suggesting a dynamic regulation of its activity based on cellular needs.
Q12: Is there a difference in the hydrolytic activity of carboxypeptidase A and B isolated from the bovine small intestine?
A12: [] Yes, while both carboxypeptidase A and B are present in the bovine small intestine, they exhibit distinct substrate preferences. Carboxypeptidase A efficiently hydrolyzes [Met]enkephalin-Arg6-Phe7 and [Leu]enkephalin but not [Met]enkephalin. Conversely, carboxypeptidase B shows specificity for [Met]enkephalin-Arg6, releasing [Met]enkephalin. This difference in substrate specificity highlights their unique roles in intestinal peptide processing.
Q13: How have computational methods been employed to understand the structure and function of human procarboxypeptidase A2?
A13: [] Researchers have used advanced molecular modeling techniques to generate a three-dimensional structure of human procarboxypeptidase A2. This model, based on the known structures of bovine carboxypeptidase A and porcine procarboxypeptidase A1, provides insights into the enzyme's specificity and activation mechanism.
Q14: How do structural modifications of inhibitors affect their potency towards carboxypeptidases A and B?
A14: [] Designing inhibitors with specific functional groups that can interact with distinct substrate-binding areas within the active sites of carboxypeptidases A and B has yielded highly potent and selective inhibitors. For instance, incorporating a sulfhydryl group, capable of binding to the zinc ion in the active site, significantly enhances the inhibitory potency. Additionally, incorporating a benzyl group in the inhibitor structure increases its selectivity towards carboxypeptidase A, while a guanidinopropyl group enhances its selectivity towards carboxypeptidase B.
Q15: How do tripeptide phosphonate inhibitors interact with Carboxypeptidase A, and what insights do they provide into the enzyme's mechanism?
A16: [] Tripeptide phosphonate inhibitors exhibit strong binding to Carboxypeptidase A. Their binding mode, elucidated through X-ray crystallography, reveals key interactions that contribute to their high affinity. The phosphinyl group of these inhibitors coordinates with the active-site zinc ion, mimicking the proposed transition state of the enzyme-catalyzed reaction.
Q16: Can molecularly imprinted polymers (MIPs) mimic the catalytic activity of carboxypeptidase A?
A17: [] Yes, MIPs, synthesized using transition state analogues (TSAs) that mimic the tetrahedral intermediate of carboxypeptidase A, have demonstrated impressive catalytic activity in hydrolyzing carbonate substrates. By incorporating catalytic elements like amidinium groups and metal ions (Zn2+ or Cu2+) in a specific orientation within the imprinted cavities, these MIPs achieve remarkable rate enhancements, reaching up to 10^5-fold compared to the uncatalyzed reactions.
Q17: What methods have been employed to study the different forms of beta-galactosidase?
A18: [] Researchers have utilized techniques like high-performance liquid chromatography (HPLC) and immunoprecipitation with specific antibodies to study and separate the different forms of beta-galactosidase, including its monomeric, dimeric, and high-molecular-weight complex forms. These methods provide insights into the composition and stability of these various forms in different disease states like GM1-gangliosidosis and beta-galactosialidosis.
Q18: What analytical techniques are employed to study the kinetic properties of Carboxypeptidase A?
A19: [, ] Researchers utilize various kinetic assays, often coupled with spectroscopic methods like absorbance or fluorescence spectroscopy, to study the catalytic activity of Carboxypeptidase A. These assays measure the rate of substrate hydrolysis under different conditions, providing information on enzyme kinetics, substrate specificity, and the impact of inhibitors. Additionally, X-ray crystallography plays a crucial role in determining the three-dimensional structures of Carboxypeptidase A in complex with substrates, inhibitors, or transition-state analogs, further elucidating the enzyme's mechanism.
Q19: How is carboxypeptidase activity measured in environmental samples like pond, river, and seawater?
A20: [] The enzymatic activity of carboxypeptidases in environmental samples is often measured using synthetic peptide substrates that mimic their natural targets. The rate of hydrolysis of these substrates, which can be monitored using techniques like spectrophotometry or fluorometry, provides a quantitative measure of the enzyme's activity in the sample.
Q20: What methods are employed to analyze and quantify mast cell proteases in human vascular tissue?
A21: [] Simultaneous analysis of mast cell proteases, including carboxypeptidase A, chymase, and tryptase, in human vascular tissue is often achieved using a combination of techniques like enzyme-linked immunosorbent assay (ELISA) and activity-based assays. ELISA allows for the specific quantification of each protease based on antibody recognition, while activity-based assays measure their enzymatic activities, providing complementary information about their presence and functionality within the tissue.
体外研究产品的免责声明和信息
请注意,BenchChem 上展示的所有文章和产品信息仅供信息参考。 BenchChem 上可购买的产品专为体外研究设计,这些研究在生物体外进行。体外研究,源自拉丁语 "in glass",涉及在受控实验室环境中使用细胞或组织进行的实验。重要的是要注意,这些产品没有被归类为药物或药品,他们没有得到 FDA 的批准,用于预防、治疗或治愈任何医疗状况、疾病或疾病。我们必须强调,将这些产品以任何形式引入人类或动物的身体都是法律严格禁止的。遵守这些指南对确保研究和实验的法律和道德标准的符合性至关重要。