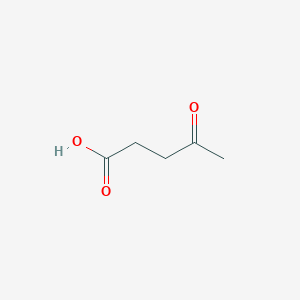
乙酰丙酸
概述
科学研究应用
左旋戊二酸在科学研究和工业中有着广泛的应用:
化学: 它被用作各种化学品的先驱,包括氨基乙酰丙酸,一种可生物降解的除草剂.
生物学: 左旋戊二酸衍生物用于合成药物和农用化学品.
工业: 左旋戊二酸用于生产增塑剂、溶剂和香料.
作用机制
左旋戊二酸通过各种分子靶标和途径发挥其作用。 例如,它可以抑制食品工业中食源性病原体的生长 . 在生物系统中,左旋戊二酸可以转化为 γ-戊内酯,它具有潜在的生物燃料应用 . 该转化涉及将酮基还原为羟基,然后环化形成内酯环 .
类似化合物:
氨基乙酰丙酸: 用作可生物降解的除草剂和光动力疗法。
双酚酸: 用于生产聚合物和树脂。
γ-戊内酯: 一种潜在的生物燃料和溶剂.
独特性: 左旋戊二酸因其作为平台化学品的通用性而独树一帜。 它可以转化为各种有价值的化学品,使其成为开发可持续和可再生化学过程的关键化合物 .
生化分析
Biochemical Properties
Levulinic acid interacts with various enzymes and proteins in biochemical reactions. It is used as a feedstock material for the synthesis of several compounds such as diphenolic acid, γ-valerolactone, various alkyl esters, and valerate . The nature of these interactions is largely dependent on the functional groups present in levulinic acid, specifically the carboxylic acid (RCOOH) and ketonic (R2C=O) groups .
Cellular Effects
The effects of levulinic acid on cells and cellular processes are complex and multifaceted. It influences cell function by interacting with various cell signaling pathways, gene expression mechanisms, and cellular metabolism processes
Molecular Mechanism
At the molecular level, levulinic acid exerts its effects through binding interactions with biomolecules, enzyme inhibition or activation, and changes in gene expression . The exact mechanism of action is dependent on the specific biomolecule or enzyme that levulinic acid is interacting with.
Temporal Effects in Laboratory Settings
In laboratory settings, the effects of levulinic acid can change over time. This includes information on the product’s stability, degradation, and any long-term effects on cellular function observed in in vitro or in vivo studies
Dosage Effects in Animal Models
The effects of levulinic acid can vary with different dosages in animal models . While specific threshold effects, toxic effects, or adverse effects at high doses have not been extensively studied, it is known that the impact of levulinic acid is dose-dependent.
Metabolic Pathways
Levulinic acid is involved in various metabolic pathways. It interacts with several enzymes and cofactors, and can influence metabolic flux or metabolite levels . The specific metabolic pathways that levulinic acid is involved in are still being researched.
Subcellular Localization
The subcellular localization of levulinic acid and its effects on activity or function are areas of active research . It is possible that there are targeting signals or post-translational modifications that direct levulinic acid to specific compartments or organelles.
准备方法
化学反应分析
反应类型: 左旋戊二酸会经历各种化学反应,包括:
氧化: 左旋戊二酸可以被氧化生成琥珀酸。
还原: 它可以被还原生成 γ-戊内酯。
常用试剂和条件:
氧化: 常见的氧化剂包括高锰酸钾和过氧化氢。
还原: 钯碳(Pd/C)等催化剂用于还原过程。
主要产品:
琥珀酸: 来自氧化。
γ-戊内酯: 来自还原。
戊二酸酯: 来自酯化.
相似化合物的比较
Aminolevulinic acid: Used as a biodegradable herbicide and in photodynamic therapy.
Diphenolic acid: Used in the production of polymers and resins.
Gamma-valerolactone: A potential biofuel and solvent.
Uniqueness: Laevulinic acid is unique due to its versatility as a platform chemical. It can be converted into a wide range of valuable chemicals, making it a key compound in the development of sustainable and renewable chemical processes .
属性
IUPAC Name |
4-oxopentanoic acid | |
---|---|---|
Source | PubChem | |
URL | https://pubchem.ncbi.nlm.nih.gov | |
Description | Data deposited in or computed by PubChem | |
InChI |
InChI=1S/C5H8O3/c1-4(6)2-3-5(7)8/h2-3H2,1H3,(H,7,8) | |
Source | PubChem | |
URL | https://pubchem.ncbi.nlm.nih.gov | |
Description | Data deposited in or computed by PubChem | |
InChI Key |
JOOXCMJARBKPKM-UHFFFAOYSA-N | |
Source | PubChem | |
URL | https://pubchem.ncbi.nlm.nih.gov | |
Description | Data deposited in or computed by PubChem | |
Canonical SMILES |
CC(=O)CCC(=O)O | |
Source | PubChem | |
URL | https://pubchem.ncbi.nlm.nih.gov | |
Description | Data deposited in or computed by PubChem | |
Molecular Formula |
C5H8O3 | |
Source | PubChem | |
URL | https://pubchem.ncbi.nlm.nih.gov | |
Description | Data deposited in or computed by PubChem | |
Related CAS |
19856-23-6 (hydrochloride salt), 591-64-0 (calcium salt) | |
Record name | Levulinic acid | |
Source | ChemIDplus | |
URL | https://pubchem.ncbi.nlm.nih.gov/substance/?source=chemidplus&sourceid=0000123762 | |
Description | ChemIDplus is a free, web search system that provides access to the structure and nomenclature authority files used for the identification of chemical substances cited in National Library of Medicine (NLM) databases, including the TOXNET system. | |
DSSTOX Substance ID |
DTXSID8021648 | |
Record name | 4-Oxopentanoic acid | |
Source | EPA DSSTox | |
URL | https://comptox.epa.gov/dashboard/DTXSID8021648 | |
Description | DSSTox provides a high quality public chemistry resource for supporting improved predictive toxicology. | |
Molecular Weight |
116.11 g/mol | |
Source | PubChem | |
URL | https://pubchem.ncbi.nlm.nih.gov | |
Description | Data deposited in or computed by PubChem | |
Physical Description |
Pellets or Large Crystals, Liquid, Solid; Product of industry is yellow; mp = 33-35 deg C; [Merck Index] Solid; mp = 30-33 deg C; [Sigma-Aldrich MSDS], Solid, yellow to brown liquid which may congeal; with mild caramellic odour | |
Record name | Pentanoic acid, 4-oxo- | |
Source | EPA Chemicals under the TSCA | |
URL | https://www.epa.gov/chemicals-under-tsca | |
Description | EPA Chemicals under the Toxic Substances Control Act (TSCA) collection contains information on chemicals and their regulations under TSCA, including non-confidential content from the TSCA Chemical Substance Inventory and Chemical Data Reporting. | |
Record name | Levulinic acid | |
Source | Haz-Map, Information on Hazardous Chemicals and Occupational Diseases | |
URL | https://haz-map.com/Agents/21110 | |
Description | Haz-Map® is an occupational health database designed for health and safety professionals and for consumers seeking information about the adverse effects of workplace exposures to chemical and biological agents. | |
Explanation | Copyright (c) 2022 Haz-Map(R). All rights reserved. Unless otherwise indicated, all materials from Haz-Map are copyrighted by Haz-Map(R). No part of these materials, either text or image may be used for any purpose other than for personal use. Therefore, reproduction, modification, storage in a retrieval system or retransmission, in any form or by any means, electronic, mechanical or otherwise, for reasons other than personal use, is strictly prohibited without prior written permission. | |
Record name | Levulinic acid | |
Source | Human Metabolome Database (HMDB) | |
URL | http://www.hmdb.ca/metabolites/HMDB0000720 | |
Description | The Human Metabolome Database (HMDB) is a freely available electronic database containing detailed information about small molecule metabolites found in the human body. | |
Explanation | HMDB is offered to the public as a freely available resource. Use and re-distribution of the data, in whole or in part, for commercial purposes requires explicit permission of the authors and explicit acknowledgment of the source material (HMDB) and the original publication (see the HMDB citing page). We ask that users who download significant portions of the database cite the HMDB paper in any resulting publications. | |
Record name | Levulinic acid | |
Source | Joint FAO/WHO Expert Committee on Food Additives (JECFA) | |
URL | https://www.fao.org/food/food-safety-quality/scientific-advice/jecfa/jecfa-flav/details/en/c/1110/ | |
Description | The flavoring agent databse provides the most recent specifications for flavorings evaluated by JECFA. | |
Explanation | Permission from WHO is not required for the use of WHO materials issued under the Creative Commons Attribution-NonCommercial-ShareAlike 3.0 Intergovernmental Organization (CC BY-NC-SA 3.0 IGO) licence. | |
Boiling Point |
245.00 to 246.00 °C. @ 760.00 mm Hg | |
Record name | Laevulinic Acid | |
Source | DrugBank | |
URL | https://www.drugbank.ca/drugs/DB02239 | |
Description | The DrugBank database is a unique bioinformatics and cheminformatics resource that combines detailed drug (i.e. chemical, pharmacological and pharmaceutical) data with comprehensive drug target (i.e. sequence, structure, and pathway) information. | |
Explanation | Creative Common's Attribution-NonCommercial 4.0 International License (http://creativecommons.org/licenses/by-nc/4.0/legalcode) | |
Record name | Levulinic acid | |
Source | Human Metabolome Database (HMDB) | |
URL | http://www.hmdb.ca/metabolites/HMDB0000720 | |
Description | The Human Metabolome Database (HMDB) is a freely available electronic database containing detailed information about small molecule metabolites found in the human body. | |
Explanation | HMDB is offered to the public as a freely available resource. Use and re-distribution of the data, in whole or in part, for commercial purposes requires explicit permission of the authors and explicit acknowledgment of the source material (HMDB) and the original publication (see the HMDB citing page). We ask that users who download significant portions of the database cite the HMDB paper in any resulting publications. | |
Solubility |
soluble in water, alcohol, and oil, 1 mL in 1 mL 95% alcohol (in ethanol) | |
Record name | Levulinic acid | |
Source | Joint FAO/WHO Expert Committee on Food Additives (JECFA) | |
URL | https://www.fao.org/food/food-safety-quality/scientific-advice/jecfa/jecfa-flav/details/en/c/1110/ | |
Description | The flavoring agent databse provides the most recent specifications for flavorings evaluated by JECFA. | |
Explanation | Permission from WHO is not required for the use of WHO materials issued under the Creative Commons Attribution-NonCommercial-ShareAlike 3.0 Intergovernmental Organization (CC BY-NC-SA 3.0 IGO) licence. | |
Density |
1.136-1.147 (20°) | |
Record name | Levulinic acid | |
Source | Joint FAO/WHO Expert Committee on Food Additives (JECFA) | |
URL | https://www.fao.org/food/food-safety-quality/scientific-advice/jecfa/jecfa-flav/details/en/c/1110/ | |
Description | The flavoring agent databse provides the most recent specifications for flavorings evaluated by JECFA. | |
Explanation | Permission from WHO is not required for the use of WHO materials issued under the Creative Commons Attribution-NonCommercial-ShareAlike 3.0 Intergovernmental Organization (CC BY-NC-SA 3.0 IGO) licence. | |
CAS No. |
123-76-2 | |
Record name | Levulinic acid | |
Source | CAS Common Chemistry | |
URL | https://commonchemistry.cas.org/detail?cas_rn=123-76-2 | |
Description | CAS Common Chemistry is an open community resource for accessing chemical information. Nearly 500,000 chemical substances from CAS REGISTRY cover areas of community interest, including common and frequently regulated chemicals, and those relevant to high school and undergraduate chemistry classes. This chemical information, curated by our expert scientists, is provided in alignment with our mission as a division of the American Chemical Society. | |
Explanation | The data from CAS Common Chemistry is provided under a CC-BY-NC 4.0 license, unless otherwise stated. | |
Record name | Levulinic acid | |
Source | ChemIDplus | |
URL | https://pubchem.ncbi.nlm.nih.gov/substance/?source=chemidplus&sourceid=0000123762 | |
Description | ChemIDplus is a free, web search system that provides access to the structure and nomenclature authority files used for the identification of chemical substances cited in National Library of Medicine (NLM) databases, including the TOXNET system. | |
Record name | Laevulinic Acid | |
Source | DrugBank | |
URL | https://www.drugbank.ca/drugs/DB02239 | |
Description | The DrugBank database is a unique bioinformatics and cheminformatics resource that combines detailed drug (i.e. chemical, pharmacological and pharmaceutical) data with comprehensive drug target (i.e. sequence, structure, and pathway) information. | |
Explanation | Creative Common's Attribution-NonCommercial 4.0 International License (http://creativecommons.org/licenses/by-nc/4.0/legalcode) | |
Record name | LEVULINIC ACID | |
Source | DTP/NCI | |
URL | https://dtp.cancer.gov/dtpstandard/servlet/dwindex?searchtype=NSC&outputformat=html&searchlist=3716 | |
Description | The NCI Development Therapeutics Program (DTP) provides services and resources to the academic and private-sector research communities worldwide to facilitate the discovery and development of new cancer therapeutic agents. | |
Explanation | Unless otherwise indicated, all text within NCI products is free of copyright and may be reused without our permission. Credit the National Cancer Institute as the source. | |
Record name | Pentanoic acid, 4-oxo- | |
Source | EPA Chemicals under the TSCA | |
URL | https://www.epa.gov/chemicals-under-tsca | |
Description | EPA Chemicals under the Toxic Substances Control Act (TSCA) collection contains information on chemicals and their regulations under TSCA, including non-confidential content from the TSCA Chemical Substance Inventory and Chemical Data Reporting. | |
Record name | 4-Oxopentanoic acid | |
Source | EPA DSSTox | |
URL | https://comptox.epa.gov/dashboard/DTXSID8021648 | |
Description | DSSTox provides a high quality public chemistry resource for supporting improved predictive toxicology. | |
Record name | 4-oxovaleric acid | |
Source | European Chemicals Agency (ECHA) | |
URL | https://echa.europa.eu/substance-information/-/substanceinfo/100.004.228 | |
Description | The European Chemicals Agency (ECHA) is an agency of the European Union which is the driving force among regulatory authorities in implementing the EU's groundbreaking chemicals legislation for the benefit of human health and the environment as well as for innovation and competitiveness. | |
Explanation | Use of the information, documents and data from the ECHA website is subject to the terms and conditions of this Legal Notice, and subject to other binding limitations provided for under applicable law, the information, documents and data made available on the ECHA website may be reproduced, distributed and/or used, totally or in part, for non-commercial purposes provided that ECHA is acknowledged as the source: "Source: European Chemicals Agency, http://echa.europa.eu/". Such acknowledgement must be included in each copy of the material. ECHA permits and encourages organisations and individuals to create links to the ECHA website under the following cumulative conditions: Links can only be made to webpages that provide a link to the Legal Notice page. | |
Record name | LEVULINIC ACID | |
Source | FDA Global Substance Registration System (GSRS) | |
URL | https://gsrs.ncats.nih.gov/ginas/app/beta/substances/RYX5QG61EI | |
Description | The FDA Global Substance Registration System (GSRS) enables the efficient and accurate exchange of information on what substances are in regulated products. Instead of relying on names, which vary across regulatory domains, countries, and regions, the GSRS knowledge base makes it possible for substances to be defined by standardized, scientific descriptions. | |
Explanation | Unless otherwise noted, the contents of the FDA website (www.fda.gov), both text and graphics, are not copyrighted. They are in the public domain and may be republished, reprinted and otherwise used freely by anyone without the need to obtain permission from FDA. Credit to the U.S. Food and Drug Administration as the source is appreciated but not required. | |
Record name | Levulinic acid | |
Source | Human Metabolome Database (HMDB) | |
URL | http://www.hmdb.ca/metabolites/HMDB0000720 | |
Description | The Human Metabolome Database (HMDB) is a freely available electronic database containing detailed information about small molecule metabolites found in the human body. | |
Explanation | HMDB is offered to the public as a freely available resource. Use and re-distribution of the data, in whole or in part, for commercial purposes requires explicit permission of the authors and explicit acknowledgment of the source material (HMDB) and the original publication (see the HMDB citing page). We ask that users who download significant portions of the database cite the HMDB paper in any resulting publications. | |
Melting Point |
30 - 33 °C | |
Record name | Laevulinic Acid | |
Source | DrugBank | |
URL | https://www.drugbank.ca/drugs/DB02239 | |
Description | The DrugBank database is a unique bioinformatics and cheminformatics resource that combines detailed drug (i.e. chemical, pharmacological and pharmaceutical) data with comprehensive drug target (i.e. sequence, structure, and pathway) information. | |
Explanation | Creative Common's Attribution-NonCommercial 4.0 International License (http://creativecommons.org/licenses/by-nc/4.0/legalcode) | |
Record name | Levulinic acid | |
Source | Human Metabolome Database (HMDB) | |
URL | http://www.hmdb.ca/metabolites/HMDB0000720 | |
Description | The Human Metabolome Database (HMDB) is a freely available electronic database containing detailed information about small molecule metabolites found in the human body. | |
Explanation | HMDB is offered to the public as a freely available resource. Use and re-distribution of the data, in whole or in part, for commercial purposes requires explicit permission of the authors and explicit acknowledgment of the source material (HMDB) and the original publication (see the HMDB citing page). We ask that users who download significant portions of the database cite the HMDB paper in any resulting publications. | |
Synthesis routes and methods I
Procedure details
Synthesis routes and methods II
Procedure details
Synthesis routes and methods III
Procedure details
Synthesis routes and methods IV
Procedure details
Synthesis routes and methods V
Procedure details
Retrosynthesis Analysis
AI-Powered Synthesis Planning: Our tool employs the Template_relevance Pistachio, Template_relevance Bkms_metabolic, Template_relevance Pistachio_ringbreaker, Template_relevance Reaxys, Template_relevance Reaxys_biocatalysis model, leveraging a vast database of chemical reactions to predict feasible synthetic routes.
One-Step Synthesis Focus: Specifically designed for one-step synthesis, it provides concise and direct routes for your target compounds, streamlining the synthesis process.
Accurate Predictions: Utilizing the extensive PISTACHIO, BKMS_METABOLIC, PISTACHIO_RINGBREAKER, REAXYS, REAXYS_BIOCATALYSIS database, our tool offers high-accuracy predictions, reflecting the latest in chemical research and data.
Strategy Settings
Precursor scoring | Relevance Heuristic |
---|---|
Min. plausibility | 0.01 |
Model | Template_relevance |
Template Set | Pistachio/Bkms_metabolic/Pistachio_ringbreaker/Reaxys/Reaxys_biocatalysis |
Top-N result to add to graph | 6 |
Feasible Synthetic Routes
Q1: What are the primary sources and methods for producing levulinic acid?
A1: Levulinic acid can be produced from renewable biomass sources, primarily lignocellulosic materials like wood, straw, and agricultural residues [, , , , , ]. The primary method involves acid-catalyzed hydrolysis of cellulose, a major component of these materials [, , , ]. This process typically involves two steps: (1) hydrolysis of cellulose to glucose and other intermediates and (2) dehydration of these intermediates to levulinic acid [].
Q2: What are the advantages of using concentrated phosphoric or sulfuric acids for levulinic acid production?
A2: Concentrated phosphoric and sulfuric acids effectively disrupt the hydrogen bonds within cellulose, promoting its breakdown into levulinic acid at lower temperatures compared to dilute acids []. This milder approach limits the formation of unwanted by-products and enhances the overall yield of levulinic acid [].
Q3: How can biphasic solvent systems enhance the production of levulinic acid from cellulose?
A3: Biphasic systems, comprising an extractive organic layer and an acidic aqueous layer, offer several advantages. First, they allow for higher concentrations of levulinic acid in the final product []. Second, by selectively partitioning levulinic acid into the organic phase, they simplify its separation and purification [, , ]. Lastly, this approach can minimize the degradation of solid acid catalysts, enhancing their reusability [].
Q4: What role does pretreatment play in the conversion of lignocellulosic biomass to levulinic acid?
A4: Pretreatment methods, such as dewaxing and delignification, help to break down the complex structure of lignocellulosic biomass, making cellulose more accessible for conversion to levulinic acid []. These methods remove lignin, a component that hinders cellulose hydrolysis, thereby improving the yield of levulinic acid [].
Q5: What are some innovative approaches being explored to enhance the efficiency of levulinic acid production from biomass?
A5: Researchers are exploring innovative approaches such as using ionic liquids as solvents [, , ]. These liquids effectively dissolve cellulose under milder conditions, promoting its conversion to levulinic acid [, ]. Additionally, microwave irradiation has been investigated as a potential method to accelerate the reaction rate and improve the yield of levulinic acid [].
Q6: Can levulinic acid be produced from sources other than lignocellulosic biomass?
A6: Yes, alternative sources like chitosan, a derivative of chitin found in crustacean shells, have shown potential for levulinic acid production []. Acidic-hydrothermal hydrolysis of chitosan has been successfully employed to produce levulinic acid, showcasing the versatility of this platform chemical [].
Q7: What are the key challenges associated with the industrial-scale production of levulinic acid from biomass?
A7: Despite its potential, several challenges hinder the large-scale production of levulinic acid. These include the high cost of pretreatment technologies, the formation of by-products like humins that complicate purification, and the need for efficient and cost-effective separation techniques [].
Q8: What are the potential applications of levulinic acid?
A8: Levulinic acid is a valuable platform chemical for various applications. It serves as a precursor for producing solvents, polymers, herbicides, fuel additives, and pharmaceuticals [, ]. Its derivatives, such as levulinate esters and γ-valerolactone, hold significant promise as biofuels and fuel additives [, , , ].
Q9: How is levulinic acid converted into γ-valerolactone (GVL)?
A9: Levulinic acid is converted to GVL via catalytic transfer hydrogenation, typically using metal-based catalysts like copper or tin supported on various materials [, ]. This reaction involves the hydrogenation of the ketone group in levulinic acid followed by intramolecular cyclization to form the lactone ring in GVL [].
Q10: What factors influence the catalytic activity and selectivity of catalysts used in GVL production from levulinic acid?
A10: Factors such as the type of metal, metal loading, support material, reaction temperature, and pressure significantly affect catalyst performance []. For instance, higher copper loading on hydroxyapatite supports was found to decrease both levulinic acid conversion and GVL selectivity, suggesting an optimal loading for optimal performance [].
Q11: How does the choice of solvent impact the catalytic conversion of levulinic acid to GVL?
A11: The choice of solvent can significantly impact the reaction rate and product distribution. Polar protic solvents, like water, can facilitate the activation of levulinic acid and enhance the selectivity towards GVL []. In contrast, aprotic solvents, like γ-valerolactone itself, might offer better solubility for certain catalysts and substrates, impacting the overall efficiency of the process [].
Q12: What are the challenges in developing stable and reusable catalysts for levulinic acid conversion?
A12: One of the main challenges is catalyst deactivation due to coke formation or sintering of metal particles during the reaction []. Researchers are actively exploring strategies like tailoring catalyst composition, optimizing reaction conditions, and developing novel catalyst regeneration techniques to overcome this limitation.
Q13: Does levulinic acid exhibit any biological activity?
A13: While not a primary focus of the provided research, some studies suggest that levulinic acid, in combination with sodium dodecyl sulfate (SDS), possesses antimicrobial properties [, , , ]. This combination has shown efficacy against various foodborne pathogens, including E. coli and Salmonella [, , , ].
Q14: How does the combination of levulinic acid and SDS exert its antimicrobial activity?
A14: The exact mechanism of action remains unclear, but it is speculated that the combination disrupts bacterial cell membranes, leading to cell death []. Levulinic acid, being an organic acid, might contribute to the disruption of the cell membrane, while SDS, a surfactant, could further enhance this effect [].
Q15: Does levulinic acid have any known impact on porphyrin metabolism in humans?
A16: Yes, research indicates that alcohol consumption can lead to the inhibition of δ-aminolevulinic acid dehydratase (ALAD), a key enzyme in the porphyrin synthesis pathway []. This inhibition can be reversed in vitro by adding zinc ions or dithiothreitol, suggesting that alcohol might interfere with the enzyme's metal cofactor binding site [].
Q16: What are the implications of ALAD inhibition by levulinic acid?
A16: While the research primarily focuses on alcohol-induced ALAD inhibition, it highlights the potential for levulinic acid to interact with enzymes involved in porphyrin metabolism. Further research is needed to understand the specific interactions between levulinic acid and ALAD and their potential implications for human health.
Q17: What analytical techniques are commonly employed to quantify levulinic acid?
A18: High-performance liquid chromatography (HPLC) is widely used for the quantification of levulinic acid [, , , , ]. This technique allows for the separation and quantification of levulinic acid from complex mixtures, enabling researchers to assess the efficiency of production processes and monitor product purity.
Q18: Are there alternative analytical methods for analyzing levulinic acid and its derivatives?
A19: Yes, gas chromatography (GC) coupled with mass spectrometry (MS) is another powerful technique for analyzing levulinic acid and its derivatives [, ]. This method provides detailed structural information and enables the identification and quantification of different compounds in complex reaction mixtures.
Q19: What spectroscopic methods are used to characterize the structure of levulinic acid and its derivatives?
A20: Nuclear magnetic resonance (NMR) spectroscopy, including both 1H and 13C NMR, is commonly used to elucidate the structure of levulinic acid and its derivatives [, ]. Infrared (IR) spectroscopy is another valuable tool for identifying functional groups and studying intermolecular interactions [, ].
Q20: What are the challenges in accurately analyzing levulinic acid in complex mixtures?
A21: The presence of other compounds with similar chemical properties, such as formic acid and furfural, can interfere with the accurate quantification of levulinic acid []. Therefore, developing selective and sensitive analytical methods is crucial for the reliable analysis of levulinic acid in complex mixtures.
Q21: Are there any concerns regarding the toxicity or biodegradability of levulinic acid and its derivatives?
A23: While generally considered less toxic than many petroleum-derived chemicals, further research is needed to comprehensively evaluate the environmental fate and potential toxicity of levulinic acid and its derivatives [, ].
Q22: What strategies can mitigate the environmental impact of levulinic acid production and utilization?
A24: Implementing sustainable practices throughout the life cycle is crucial. This includes utilizing non-edible biomass feedstocks, optimizing production processes to minimize waste and energy consumption, and developing efficient catalyst regeneration and recycling technologies [, ].
体外研究产品的免责声明和信息
请注意,BenchChem 上展示的所有文章和产品信息仅供信息参考。 BenchChem 上可购买的产品专为体外研究设计,这些研究在生物体外进行。体外研究,源自拉丁语 "in glass",涉及在受控实验室环境中使用细胞或组织进行的实验。重要的是要注意,这些产品没有被归类为药物或药品,他们没有得到 FDA 的批准,用于预防、治疗或治愈任何医疗状况、疾病或疾病。我们必须强调,将这些产品以任何形式引入人类或动物的身体都是法律严格禁止的。遵守这些指南对确保研究和实验的法律和道德标准的符合性至关重要。