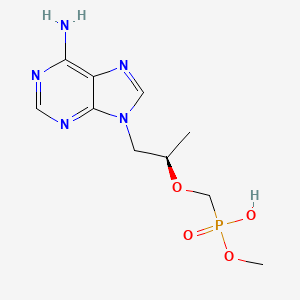
Tenofovir Monomethyl Ester
描述
Acyclic phosphonate nucleotide analogue; reverse transcriptase inhibitor. Used as an anti-HIV agent. Antiviral.
科学研究应用
Pharmacological Properties
Mechanism of Action
Tenofovir monomethyl ester acts as a nucleotide reverse transcriptase inhibitor, which is crucial for inhibiting viral replication. It is a metabolite of tenofovir disoproxil fumarate and plays a role in the pharmacokinetics of tenofovir by enhancing its bioavailability and cellular uptake.
Bioavailability Improvement
Research indicates that this compound has improved oral bioavailability compared to its parent compound, tenofovir disoproxil fumarate. Studies have shown that formulations incorporating esterase and efflux inhibitors can significantly enhance the transport and absorption of tenofovir, leading to better therapeutic outcomes in patients .
Clinical Applications
HIV Treatment and Prevention
this compound is primarily utilized in the context of HIV treatment. It is often part of combination therapies that include other antiretroviral agents. Its effectiveness has been demonstrated in various clinical trials, where it contributed to viral suppression in HIV-positive patients . Moreover, it is used in pre-exposure prophylaxis (PrEP) for individuals at high risk of HIV infection, showcasing its preventive capabilities .
Hepatitis B Treatment
The compound is also indicated for chronic hepatitis B treatment. Its antiviral efficacy against HBV has been well-documented, making it a preferred choice in managing this infection alongside HIV .
Case Studies
Several case studies have highlighted the effectiveness of this compound in clinical settings:
- Case Study on HIV Management : A patient with HIV undergoing treatment with tenofovir disoproxil fumarate demonstrated significant viral load reduction after switching to a regimen that included this compound. The switch resulted in better tolerability and fewer side effects compared to previous treatments .
- Impact on Hepatitis B Patients : In a cohort study involving patients with chronic hepatitis B, those treated with this compound showed marked improvements in liver function tests and viral load reduction after six months of therapy .
Research Findings
Recent studies have focused on the pharmacokinetics and efficacy of this compound:
- Enhanced Cellular Uptake : Research indicates that this compound achieves higher concentrations within lymphoid cells compared to tenofovir disoproxil fumarate. This enhanced cellular loading may contribute to its effectiveness as an antiviral agent .
- Pharmacokinetic Studies : A study measuring plasma levels of this compound post-administration revealed peak concentrations occurring rapidly, suggesting efficient absorption and distribution within the body .
Comparative Efficacy
Compound | Indication | Efficacy | Bioavailability |
---|---|---|---|
This compound | HIV, HBV | High viral suppression rates | Improved compared to TDF |
Tenofovir Disoproxil Fumarate | HIV, HBV | Effective but lower cellular uptake | Standard bioavailability |
Tenofovir Alafenamide Fumarate | HIV | Higher efficacy with fewer side effects | Enhanced bioavailability |
常见问题
Basic Research Questions
Q. What enzymatic pathways are critical for the metabolic activation of Tenofovir Monomethyl Ester prodrugs, and how are these assessed experimentally?
this compound prodrugs require enzymatic hydrolysis by carboxylesterases to release the active metabolite, tenofovir. Researchers typically use in vitro assays with liver microsomes or recombinant enzymes to quantify hydrolysis rates. For example, nonspecific carboxylesterases mediate the cleavage of ester bonds, followed by spontaneous degradation of intermediates like carbonic acid monomethyl ester . Kinetic parameters (e.g., Vmax, Km) are measured via HPLC or LC-MS to track metabolite formation . Preclinical models (e.g., rodent hepatocytes) further validate these pathways .
Q. How is this compound synthesized and characterized in laboratory settings?
Synthesis involves esterification of tenofovir with methyl groups under controlled acidic conditions to avoid premature hydrolysis. Key steps include:
- Protecting reactive hydroxyl groups to ensure regioselective ester formation .
- Purification via column chromatography or recrystallization. Characterization employs NMR (for structural confirmation), HPLC (purity >95%), and mass spectrometry (molecular weight verification) . Stability studies under varying pH and temperature conditions ensure compound integrity during storage .
Q. What analytical methods are recommended for quantifying this compound and its metabolites in biological matrices?
Reverse-phase HPLC with UV detection (210–220 nm) is standard for separating this compound from metabolites like tenofovir. For chiral analysis, chiral columns (e.g., Chiralcel OJ-H) resolve stereoisomers, critical for assessing enantiomeric purity . LC-MS/MS provides higher sensitivity for low-concentration samples (e.g., intracellular tenofovir diphosphate) . Method validation includes linearity (1–100 μg/mL), recovery rates (>90%), and precision (CV <10%) .
Q. How do researchers evaluate the pharmacokinetic (PK) profile of this compound in preclinical models?
PK studies in rodents or primates measure plasma concentration-time curves after oral/intravenous administration. Key parameters include AUC, Cmax, and half-life. Compartmental modeling distinguishes prodrug absorption from tenofovir distribution. Intracellular PK (e.g., tenofovir diphosphate levels in peripheral blood mononuclear cells) is quantified via LC-MS to assess antiviral efficacy .
Advanced Research Questions
Q. How can structural modifications of Tenofovir ester prodrugs enhance metabolic stability and target tissue delivery?
Modifying the ester side chain (e.g., substituting isopropyl with amide groups) alters hydrolysis rates and tissue specificity. For example, tenofovir alafenamide (TAF) uses a phenylalanine ester to improve lymphatic uptake, reducing systemic exposure. Computational docking studies predict enzyme-substrate interactions, guiding rational design . In vivo imaging (e.g., radiolabeled prodrugs) tracks tissue distribution .
Q. What experimental strategies resolve contradictions in reported antiviral efficacy of this compound across cell lines?
Discrepancies often arise from variations in cell-specific esterase activity or efflux transporters (e.g., MRP4). Researchers should:
- Normalize data to intracellular tenofovir diphosphate levels via LC-MS .
- Use CRISPR-edited cell lines to knockout specific transporters/enzymes and isolate contributing factors .
- Compare results across multiple models (e.g., primary hepatocytes vs. immortalized lines) to identify context-dependent effects .
Q. How can synthesis of this compound be optimized for scalable production while maintaining enantiomeric purity?
Flow chemistry reduces side reactions by controlling reaction time and temperature. Chiral catalysts (e.g., lipases) improve stereoselective esterification . Process analytical technology (PAT) monitors real-time purity via inline HPLC . For industrial-scale applications, crystallization conditions (e.g., solvent polarity, cooling rates) are optimized to minimize racemization .
Q. What computational tools are effective in predicting the metabolic fate of this compound prodrugs?
Molecular dynamics (MD) simulations (e.g., Amber ff14SB force field) model esterase binding and hydrolysis mechanisms. Tools like AutoDock Vina predict binding affinities for esterase variants . Systems pharmacology models integrate in vitro kinetic data to simulate in vivo PK profiles, reducing animal testing .
Q. How do researchers design in vitro models to study hepatocellular metabolism rebalancing by Tenofovir esters?
3D hepatocyte spheroids or organ-on-chip systems mimic in vivo liver zonation. Metabolomic profiling (via UPLC-QTOF-MS) tracks changes in glycolysis, oxidative phosphorylation, and lipid metabolism after prodrug exposure. RNA-seq identifies upregulated/downregulated pathways (e.g., mitochondrial biogenesis) .
属性
IUPAC Name |
[(2R)-1-(6-aminopurin-9-yl)propan-2-yl]oxymethyl-methoxyphosphinic acid | |
---|---|---|
Source | PubChem | |
URL | https://pubchem.ncbi.nlm.nih.gov | |
Description | Data deposited in or computed by PubChem | |
InChI |
InChI=1S/C10H16N5O4P/c1-7(19-6-20(16,17)18-2)3-15-5-14-8-9(11)12-4-13-10(8)15/h4-5,7H,3,6H2,1-2H3,(H,16,17)(H2,11,12,13)/t7-/m1/s1 | |
Source | PubChem | |
URL | https://pubchem.ncbi.nlm.nih.gov | |
Description | Data deposited in or computed by PubChem | |
InChI Key |
QNIIFMBLEIMHIO-SSDOTTSWSA-N | |
Source | PubChem | |
URL | https://pubchem.ncbi.nlm.nih.gov | |
Description | Data deposited in or computed by PubChem | |
Canonical SMILES |
CC(CN1C=NC2=C(N=CN=C21)N)OCP(=O)(O)OC | |
Source | PubChem | |
URL | https://pubchem.ncbi.nlm.nih.gov | |
Description | Data deposited in or computed by PubChem | |
Isomeric SMILES |
C[C@H](CN1C=NC2=C(N=CN=C21)N)OCP(=O)(O)OC | |
Source | PubChem | |
URL | https://pubchem.ncbi.nlm.nih.gov | |
Description | Data deposited in or computed by PubChem | |
Molecular Formula |
C10H16N5O4P | |
Source | PubChem | |
URL | https://pubchem.ncbi.nlm.nih.gov | |
Description | Data deposited in or computed by PubChem | |
DSSTOX Substance ID |
DTXSID00858427 | |
Record name | Methyl hydrogen ({[(2R)-1-(6-amino-9H-purin-9-yl)propan-2-yl]oxy}methyl)phosphonate | |
Source | EPA DSSTox | |
URL | https://comptox.epa.gov/dashboard/DTXSID00858427 | |
Description | DSSTox provides a high quality public chemistry resource for supporting improved predictive toxicology. | |
Molecular Weight |
301.24 g/mol | |
Source | PubChem | |
URL | https://pubchem.ncbi.nlm.nih.gov | |
Description | Data deposited in or computed by PubChem | |
CAS No. |
123155-85-1 | |
Record name | Methyl hydrogen ({[(2R)-1-(6-amino-9H-purin-9-yl)propan-2-yl]oxy}methyl)phosphonate | |
Source | EPA DSSTox | |
URL | https://comptox.epa.gov/dashboard/DTXSID00858427 | |
Description | DSSTox provides a high quality public chemistry resource for supporting improved predictive toxicology. | |
Retrosynthesis Analysis
AI-Powered Synthesis Planning: Our tool employs the Template_relevance Pistachio, Template_relevance Bkms_metabolic, Template_relevance Pistachio_ringbreaker, Template_relevance Reaxys, Template_relevance Reaxys_biocatalysis model, leveraging a vast database of chemical reactions to predict feasible synthetic routes.
One-Step Synthesis Focus: Specifically designed for one-step synthesis, it provides concise and direct routes for your target compounds, streamlining the synthesis process.
Accurate Predictions: Utilizing the extensive PISTACHIO, BKMS_METABOLIC, PISTACHIO_RINGBREAKER, REAXYS, REAXYS_BIOCATALYSIS database, our tool offers high-accuracy predictions, reflecting the latest in chemical research and data.
Strategy Settings
Precursor scoring | Relevance Heuristic |
---|---|
Min. plausibility | 0.01 |
Model | Template_relevance |
Template Set | Pistachio/Bkms_metabolic/Pistachio_ringbreaker/Reaxys/Reaxys_biocatalysis |
Top-N result to add to graph | 6 |
Feasible Synthetic Routes
体外研究产品的免责声明和信息
请注意,BenchChem 上展示的所有文章和产品信息仅供信息参考。 BenchChem 上可购买的产品专为体外研究设计,这些研究在生物体外进行。体外研究,源自拉丁语 "in glass",涉及在受控实验室环境中使用细胞或组织进行的实验。重要的是要注意,这些产品没有被归类为药物或药品,他们没有得到 FDA 的批准,用于预防、治疗或治愈任何医疗状况、疾病或疾病。我们必须强调,将这些产品以任何形式引入人类或动物的身体都是法律严格禁止的。遵守这些指南对确保研究和实验的法律和道德标准的符合性至关重要。