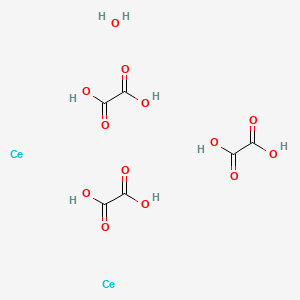
草酸铈(III)
描述
Cerium(III) oxalate, also known as cerous oxalate, is the inorganic cerium salt of oxalic acid . It is a white crystalline solid with the chemical formula of Ce2(C2O4)3 . It is used as an antiemetic and has been identified as part of the invisible ink used by Stasi operatives during the Cold War .
Synthesis Analysis
Cerium(III) oxalate can be synthesized by the reaction of oxalic acid with cerium(III) chloride . A study has shown that cerium oxalate hydrate particles were synthesized by a precipitation technique under an external magnetic field . The particle morphologies were investigated using scanning electron microscopy (SEM) and transmission electron microscope (TEM) .Molecular Structure Analysis
The molecular formula of Cerium(III) oxalate is C6Ce2O12 . Its average mass is 544.289 Da and its monoisotopic mass is 543.749817 Da .Chemical Reactions Analysis
Cerium(III) oxalate is characterized chemically by having two valence states, the +3 cerous and +4 ceric states . The ceric state is the only non-trivalent rare earth ion stable in aqueous solutions .Physical And Chemical Properties Analysis
Cerium(III) oxalate is a white crystalline solid . It is slightly soluble in water . The flash point is 188.8 °C .科学研究应用
Synthesis of Rare Earth Oxides
The compound serves as a precursor for synthesizing rare earth oxides, which are essential in various high-tech applications. The controlled thermal decomposition of Cerium(III) oxalate hydrate leads to the formation of rare earth oxides with specific sizes and shapes, influencing the final properties of the materials .
Anti-Emetic Medication
Cerium(III) oxalate has been used as an antiemetic, which is a substance that helps prevent vomiting. It has been utilized in medical treatments to alleviate symptoms of nausea and motion sickness .
安全和危害
Cerium(III) oxalate is corrosive, irritant, respiratory irritant, and toxic . It irritates skin and mucous membranes, and is a strong irritant to eyes . If it gets into the eyes, there is a danger of severe eye injury . Oxalates are corrosive to tissue and are powerful irritants. They have a caustic effect on the linings of the digestive tracts and can cause kidney damage .
作用机制
Target of Action
Cerium(III) oxalate hydrate, also known as Cerium(III) oxalate, is an inorganic cerium salt of oxalic acid It is used as an antiemetic , suggesting that it may interact with receptors or enzymes involved in nausea and vomiting.
Mode of Action
It is known that cerium salts can increase the blood coagulation rate
Biochemical Pathways
Given its role in increasing the blood coagulation rate , it may be inferred that it affects the coagulation cascade, a complex series of reactions that lead to the formation of a clot.
Pharmacokinetics
It is known to be slightly soluble in water , which could impact its absorption and distribution
Result of Action
It is known that cerium salts can cause sensitivity to heat . Additionally, oxalates are corrosive to tissue and are powerful irritants. They have a caustic effect on the linings of the digestive tracts and can cause kidney damage .
Action Environment
Environmental factors can influence the action, efficacy, and stability of Cerium(III) oxalate hydrate. For instance, the presence of an oxidizing acid solution can facilitate the oxidation of Cerium(III) ions to Cerium(IV) ions . Additionally, Cerium(III) oxalate hydrate is known to convert to the oxide when heated , which could impact its stability and efficacy.
属性
IUPAC Name |
cerium;oxalic acid;hydrate | |
---|---|---|
Source | PubChem | |
URL | https://pubchem.ncbi.nlm.nih.gov | |
Description | Data deposited in or computed by PubChem | |
InChI |
InChI=1S/3C2H2O4.2Ce.H2O/c3*3-1(4)2(5)6;;;/h3*(H,3,4)(H,5,6);;;1H2 | |
Source | PubChem | |
URL | https://pubchem.ncbi.nlm.nih.gov | |
Description | Data deposited in or computed by PubChem | |
InChI Key |
OGGMXARXHWPYSA-UHFFFAOYSA-N | |
Source | PubChem | |
URL | https://pubchem.ncbi.nlm.nih.gov | |
Description | Data deposited in or computed by PubChem | |
Canonical SMILES |
C(=O)(C(=O)O)O.C(=O)(C(=O)O)O.C(=O)(C(=O)O)O.O.[Ce].[Ce] | |
Source | PubChem | |
URL | https://pubchem.ncbi.nlm.nih.gov | |
Description | Data deposited in or computed by PubChem | |
Molecular Formula |
C6H8Ce2O13 | |
Source | PubChem | |
URL | https://pubchem.ncbi.nlm.nih.gov | |
Description | Data deposited in or computed by PubChem | |
Molecular Weight |
568.35 g/mol | |
Source | PubChem | |
URL | https://pubchem.ncbi.nlm.nih.gov | |
Description | Data deposited in or computed by PubChem | |
Physical Description |
Hygroscopic powder; [Sigma-Aldrich MSDS] | |
Record name | Cerium(III) oxalate hydrate | |
Source | Haz-Map, Information on Hazardous Chemicals and Occupational Diseases | |
URL | https://haz-map.com/Agents/20415 | |
Description | Haz-Map® is an occupational health database designed for health and safety professionals and for consumers seeking information about the adverse effects of workplace exposures to chemical and biological agents. | |
Explanation | Copyright (c) 2022 Haz-Map(R). All rights reserved. Unless otherwise indicated, all materials from Haz-Map are copyrighted by Haz-Map(R). No part of these materials, either text or image may be used for any purpose other than for personal use. Therefore, reproduction, modification, storage in a retrieval system or retransmission, in any form or by any means, electronic, mechanical or otherwise, for reasons other than personal use, is strictly prohibited without prior written permission. | |
Product Name |
Cerium;oxalic acid;hydrate | |
CAS RN |
15750-47-7 | |
Record name | Tris[oxalate(2-)]dicerium hydrate | |
Source | European Chemicals Agency (ECHA) | |
URL | https://echa.europa.eu/information-on-chemicals | |
Description | The European Chemicals Agency (ECHA) is an agency of the European Union which is the driving force among regulatory authorities in implementing the EU's groundbreaking chemicals legislation for the benefit of human health and the environment as well as for innovation and competitiveness. | |
Explanation | Use of the information, documents and data from the ECHA website is subject to the terms and conditions of this Legal Notice, and subject to other binding limitations provided for under applicable law, the information, documents and data made available on the ECHA website may be reproduced, distributed and/or used, totally or in part, for non-commercial purposes provided that ECHA is acknowledged as the source: "Source: European Chemicals Agency, http://echa.europa.eu/". Such acknowledgement must be included in each copy of the material. ECHA permits and encourages organisations and individuals to create links to the ECHA website under the following cumulative conditions: Links can only be made to webpages that provide a link to the Legal Notice page. | |
Q & A
Q1: What are the typical morphological features of cerium(III) oxalate?
A1: Cerium(III) oxalate can be synthesized into various morphologies, including nanorods and spherical nanoparticles. The oxidation state of the cerium ion plays a role in determining the final morphology. For instance, zinc(II) oxalate forms nanorods approximately 120 nm in diameter and 600 nm long, while cerium(III) oxalate forms spherical particles around 4-6 nm in size. []
Q2: How does the thermal decomposition of cerium(III) oxalate proceed?
A2: Thermal decomposition of cerium(III) oxalate can lead to the formation of cerium dioxide (CeO2). This process can yield different morphologies of cerium dioxide, including a mixture of nanorods and nanoparticles. The size of the resulting CeO2 nanoparticles can vary, with examples such as 3-4 nm for those derived from zirconium oxalate precursors. [] The decomposition temperature and heating rate can influence the final properties of the CeO2. []
Q3: Can cerium(III) oxalate be used to synthesize mixed oxides?
A3: Yes, cerium(III) oxalate can be used as a precursor for the synthesis of mixed oxides, such as uranium-cerium oxide solid solutions (U1-xCexO2+δ·nH2O). This is particularly relevant as cerium can act as a surrogate for plutonium in these materials. Hydrothermal treatment of mixed uranium(IV)-cerium(III) oxalates allows for the formation of these mixed oxides with a fluorite-type structure. []
Q4: What factors influence the hydrothermal conversion of mixed uranium(IV)-cerium(III) oxalates?
A4: The initial pH of the reaction media and the duration of hydrothermal treatment significantly affect the morphology and homogeneity of the resulting mixed oxides. For example, acidic conditions (pH ≤ 2) favor the formation of microspheres, while alkaline conditions lead to agglomerates of nanocrystallites. Longer hydrothermal treatment times generally lead to more homogeneous cationic distributions within the mixed oxides. []
Q5: What is unusual about the dehydration of cerium(III) oxalate?
A5: Unlike other rare earth oxalates, which can be dehydrated in vacuo at 320 °C without decomposing, cerium(III) oxalate converts to cerium dioxide under these conditions when exposed to air. This atypical behavior is attributed to the susceptibility of Ce(III) to oxidation to its quadrivalent state (Ce(IV)). This characteristic is also observed in plutonium(III) and americium(III) oxalates. []
Q6: What role can cerium play in the Briggs-Rauscher oscillating reaction?
A6: Cerium(III) can replace manganese(II) as a catalyst in the Briggs-Rauscher oscillating reaction, which also involves acid, iodate, hydrogen peroxide, and malonic acid. Notably, using sulfuric acid is important as cerium(III) iodate precipitates in the presence of perchloric acid. Although cerium(III) oxalate may precipitate by the reaction's end, Ce(III) proves to be a more effective catalyst at low concentrations compared to Mn(II). []
Q7: Can cerium be recovered from industrial processes?
A7: Yes, cerium can be recovered from industrial waste streams, such as those generated during brewery wastewater treatment using cerium(III) chloride as a coagulant. The sewage sludge generated in this process can be treated with hydrochloric acid to extract cerium and phosphorus. Subsequent treatment with oxalic acid precipitates cerium(III) oxalate, which can be further processed to obtain cerium oxide (CeO2) and ultimately regenerated into cerium(III) chloride. []
Q8: How is the solubility of cerium(III) oxalate determined?
A8: The solubility of cerium(III) oxalate in water can be accurately determined using radiometric methods. These methods, which involve using a radioactively labeled form of cerium(III) oxalate, have been shown to provide results consistent with other techniques, such as chemisorption on cation exchange paper followed by X-ray fluorescence analysis. These findings support the reliability of radiometric methods for solubility measurements. []
体外研究产品的免责声明和信息
请注意,BenchChem 上展示的所有文章和产品信息仅供信息参考。 BenchChem 上可购买的产品专为体外研究设计,这些研究在生物体外进行。体外研究,源自拉丁语 "in glass",涉及在受控实验室环境中使用细胞或组织进行的实验。重要的是要注意,这些产品没有被归类为药物或药品,他们没有得到 FDA 的批准,用于预防、治疗或治愈任何医疗状况、疾病或疾病。我们必须强调,将这些产品以任何形式引入人类或动物的身体都是法律严格禁止的。遵守这些指南对确保研究和实验的法律和道德标准的符合性至关重要。